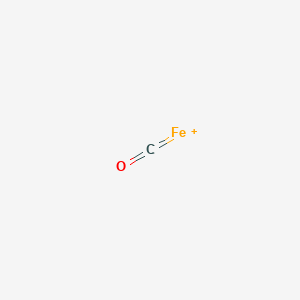
Iron(1+), carbonyl-
- Click on QUICK INQUIRY to receive a quote from our team of experts.
- With the quality product at a COMPETITIVE price, you can focus more on your research.
Overview
Description
Iron(1+), carbonyl- is a coordination complex of iron with carbon monoxide ligands. It is a member of the broader class of metal carbonyls, which are compounds consisting of a metal center bonded to carbon monoxide ligands. These compounds are significant in organometallic chemistry due to their unique bonding properties and reactivity .
Preparation Methods
Synthetic Routes and Reaction Conditions
Iron(1+), carbonyl- can be synthesized through the direct reaction of iron with carbon monoxide. The reaction typically requires high pressure and temperature to facilitate the formation of the complex. For example, iron pentacarbonyl (Fe(CO)5) can be prepared by reacting finely divided iron powder with carbon monoxide at 200 atm and 200°C .
Industrial Production Methods
Industrial production of iron carbonyl compounds often involves similar high-pressure and high-temperature conditions. Specialized equipment made of chemically resistant materials, such as copper-silver alloys, is used to handle the reactive intermediates and products .
Chemical Reactions Analysis
Types of Reactions
Iron(1+), carbonyl- undergoes various types of chemical reactions, including:
Oxidation: Reacts with oxygen to form iron oxides and carbon dioxide.
Reduction: Can reduce organic compounds, such as converting nitrobenzene to aniline.
Substitution: Carbon monoxide ligands can be replaced by other ligands, such as phosphines or alkenes
Common Reagents and Conditions
Common reagents used in reactions with iron carbonyls include halogens, acids, and other ligands. For example, reactions with halogens yield iron halides, while reactions with concentrated acids produce iron salts with the evolution of carbon monoxide .
Major Products
Major products formed from these reactions include iron halides, iron oxides, and various substituted iron carbonyl complexes .
Scientific Research Applications
Magnetic Materials
Carbonyl iron is extensively used in the production of soft magnetic materials due to its high magnetic permeability and low coercivity. These properties enhance the efficiency of electronic components such as inductors and transformers.
Application | Details |
---|---|
Inductors | Used in broadband inductors for high-power applications. |
Transformers | Improves efficiency in high-frequency transformers due to low core losses. |
Electromagnetic Interference Shielding | Utilized in EMI shielding to protect sensitive electronic devices from interference. |
Powder Metallurgy
In powder metallurgy, carbonyl iron serves as a raw material for manufacturing components with precise shapes and high density.
Product Type | Applications |
---|---|
Automotive Parts | Sintered parts such as gears and bearings for automotive applications. |
Complex Components | Used in metal injection molding processes for creating intricate shapes in various industries. |
Healthcare Applications
Carbonyl iron has gained attention in the healthcare sector, particularly for its use in treating iron deficiency anemia.
- A study indicated that carbonyl iron has a bioavailability of about 70% compared to ferrous sulfate, making it an effective supplement for individuals suffering from iron deficiency .
- Clinical trials have demonstrated its efficacy in correcting anemia with minimal side effects, thus providing a safer alternative to traditional iron salts .
Environmental Applications
Carbonyl iron is employed in environmental technologies, notably in magnetorheological fluids.
Application | Details |
---|---|
Magnetorheological Fluids | Used in applications where controlled viscosity is required, such as dampers and clutches. |
Catalysis
Due to its high surface area and reactivity, carbonyl iron acts as a catalyst in various chemical reactions.
- It is particularly useful in hydrogenation reactions within petrochemical and pharmaceutical industries .
Food Fortification
Carbonyl iron is also utilized in food products for fortification purposes to combat iron deficiency anemia.
Case Study 1: Use in Electronics
A study highlighted the application of carbonyl iron powder in the manufacturing of radio frequency equipment during World War II. The powder's properties allowed for the production of low hysteresis induction coils suitable for carrier wave frequencies over 100 MHz, demonstrating its critical role in military technology .
Case Study 2: Health Benefits
A randomized double-blind study assessed the bioavailability of carbonyl iron compared to traditional supplements. Results indicated that participants taking carbonyl iron experienced significant increases in serum ferritin levels without severe side effects, showcasing its potential as a safer alternative for treating anemia .
Case Study 3: Magnetorheological Fluids
Research into magnetorheological fluids containing carbonyl iron revealed their effectiveness in automotive applications, particularly in improving vehicle stability through controlled damping systems .
Mechanism of Action
The mechanism of action of iron(1+), carbonyl- involves its ability to donate and accept electrons through its carbon monoxide ligands. This electron transfer capability allows it to participate in various catalytic processes and chemical reactions. The compound’s molecular targets include other metal centers and organic molecules, where it can facilitate bond formation and cleavage .
Comparison with Similar Compounds
Iron(1+), carbonyl- can be compared with other metal carbonyls, such as:
Chromium hexacarbonyl (Cr(CO)6): Similar in structure but with chromium as the central metal.
Nickel tetracarbonyl (Ni(CO)4): Contains nickel and has a tetrahedral geometry.
Diiron nonacarbonyl (Fe2(CO)9): A dimeric form with two iron centers and nine carbon monoxide ligands .
These compounds share similar bonding properties and reactivity but differ in their metal centers and coordination geometries, which influence their specific applications and reactivity patterns.
Properties
CAS No. |
35038-14-3 |
---|---|
Molecular Formula |
CFeO+ |
Molecular Weight |
83.85 g/mol |
InChI |
InChI=1S/CO.Fe/c1-2;/q;+1 |
InChI Key |
FGGXMJUBBBCAOD-UHFFFAOYSA-N |
Canonical SMILES |
C(=O)=[Fe+] |
Origin of Product |
United States |
Disclaimer and Information on In-Vitro Research Products
Please be aware that all articles and product information presented on BenchChem are intended solely for informational purposes. The products available for purchase on BenchChem are specifically designed for in-vitro studies, which are conducted outside of living organisms. In-vitro studies, derived from the Latin term "in glass," involve experiments performed in controlled laboratory settings using cells or tissues. It is important to note that these products are not categorized as medicines or drugs, and they have not received approval from the FDA for the prevention, treatment, or cure of any medical condition, ailment, or disease. We must emphasize that any form of bodily introduction of these products into humans or animals is strictly prohibited by law. It is essential to adhere to these guidelines to ensure compliance with legal and ethical standards in research and experimentation.