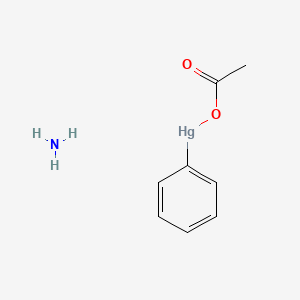
Phenylmercuric ammonium acetate
- Click on QUICK INQUIRY to receive a quote from our team of experts.
- With the quality product at a COMPETITIVE price, you can focus more on your research.
Overview
Description
Phenylmercuric ammonium acetate is an organomercurial compound known for its antimicrobial properties. It has been widely used as a preservative, fungicide, and slimicide. The compound is characterized by the presence of a phenyl group attached to a mercury atom, which is further bonded to an ammonium acetate group. This unique structure imparts significant biological activity, making it useful in various applications .
Preparation Methods
Synthetic Routes and Reaction Conditions: Phenylmercuric ammonium acetate can be synthesized through the reaction of phenylmercuric acetate with ammonium acetate. The reaction typically occurs in an aqueous medium under controlled temperature and pH conditions to ensure the formation of the desired product.
Industrial Production Methods: Industrial production of this compound involves large-scale synthesis using similar reaction conditions as in the laboratory. The process is optimized for yield and purity, often involving purification steps such as recrystallization or distillation to remove impurities .
Chemical Reactions Analysis
Types of Reactions: Phenylmercuric ammonium acetate undergoes various chemical reactions, including:
Oxidation: The compound can be oxidized to form phenylmercuric hydroxide.
Reduction: Reduction reactions can convert it to phenylmercuric chloride.
Substitution: It can participate in substitution reactions where the acetate group is replaced by other functional groups.
Common Reagents and Conditions:
Oxidation: Common oxidizing agents include hydrogen peroxide and potassium permanganate.
Reduction: Reducing agents such as sodium borohydride are used.
Substitution: Reagents like halides and other nucleophiles are employed under mild to moderate conditions.
Major Products:
Oxidation: Phenylmercuric hydroxide.
Reduction: Phenylmercuric chloride.
Substitution: Various phenylmercuric derivatives depending on the substituent introduced.
Scientific Research Applications
Phenylmercuric ammonium acetate has diverse applications in scientific research:
Chemistry: Used as a catalyst in organic synthesis and as a reagent in analytical chemistry.
Biology: Employed in the study of enzyme inhibition and protein interactions.
Medicine: Historically used as a preservative in ophthalmic solutions and other pharmaceutical formulations.
Industry: Utilized as a fungicide in agriculture and as a preservative in paints and coatings.
Mechanism of Action
The antimicrobial activity of phenylmercuric ammonium acetate is primarily due to its ability to disrupt microbial cell membranes and inhibit essential enzymatic functions. The mercury atom interacts with thiol groups in proteins, leading to the inactivation of enzymes and disruption of cellular processes. This results in the death of microbial cells .
Comparison with Similar Compounds
- Phenylmercuric acetate
- Phenylmercuric nitrate
- Thimerosal
Comparison: Phenylmercuric ammonium acetate is unique due to its specific ammonium acetate group, which imparts distinct solubility and reactivity properties compared to other phenylmercuric compounds. For instance, phenylmercuric acetate is more commonly used as a fungicide, while thimerosal is widely known for its use as a preservative in vaccines .
Properties
CAS No. |
34604-38-1 |
---|---|
Molecular Formula |
C8H11HgNO2 |
Molecular Weight |
353.77 g/mol |
IUPAC Name |
acetyloxy(phenyl)mercury;azane |
InChI |
InChI=1S/C6H5.C2H4O2.Hg.H3N/c1-2-4-6-5-3-1;1-2(3)4;;/h1-5H;1H3,(H,3,4);;1H3/q;;+1;/p-1 |
InChI Key |
LPDJEBDOSVRHKE-UHFFFAOYSA-M |
Canonical SMILES |
CC(=O)O[Hg]C1=CC=CC=C1.N |
Origin of Product |
United States |
Disclaimer and Information on In-Vitro Research Products
Please be aware that all articles and product information presented on BenchChem are intended solely for informational purposes. The products available for purchase on BenchChem are specifically designed for in-vitro studies, which are conducted outside of living organisms. In-vitro studies, derived from the Latin term "in glass," involve experiments performed in controlled laboratory settings using cells or tissues. It is important to note that these products are not categorized as medicines or drugs, and they have not received approval from the FDA for the prevention, treatment, or cure of any medical condition, ailment, or disease. We must emphasize that any form of bodily introduction of these products into humans or animals is strictly prohibited by law. It is essential to adhere to these guidelines to ensure compliance with legal and ethical standards in research and experimentation.