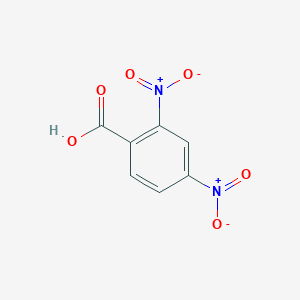
2,4-Dinitrobenzoic acid
Overview
Description
2,4-Dinitrobenzoic acid is an organic compound with the molecular formula C₇H₄N₂O₆. It is a derivative of benzoic acid, where two nitro groups are substituted at the 2 and 4 positions of the benzene ring. This compound is known for its yellow crystalline appearance and is used in various chemical applications due to its reactive nature.
Preparation Methods
Synthetic Routes and Reaction Conditions: 2,4-Dinitrobenzoic acid can be synthesized through the nitration of benzoic acid. The process involves the following steps:
Nitration: Benzoic acid is treated with a mixture of concentrated nitric acid and sulfuric acid. The reaction is typically carried out at a controlled temperature to avoid over-nitration.
Purification: The resulting product is purified through recrystallization from a suitable solvent, such as methanol or water, to obtain pure this compound.
Industrial Production Methods: In industrial settings, the production of this compound may involve the nitration of bibenzyl followed by oxidation. This method provides a more efficient route with higher yields and easier purification steps .
Chemical Reactions Analysis
2,4-Dinitrobenzoic acid undergoes various chemical reactions, including:
Reduction: The nitro groups can be reduced to amino groups using reducing agents such as tin and hydrochloric acid or catalytic hydrogenation.
Substitution: The nitro groups make the aromatic ring more reactive towards nucleophilic substitution reactions. For example, it can react with nucleophiles like amines to form substituted derivatives.
Decarboxylation: Under certain conditions, this compound can undergo decarboxylation to form 2,4-dinitrobenzene.
Common reagents and conditions used in these reactions include reducing agents like tin and hydrochloric acid, nucleophiles like amines, and catalysts for hydrogenation reactions. The major products formed from these reactions include amino derivatives and substituted aromatic compounds.
Scientific Research Applications
2,4-Dinitrobenzoic acid has several applications in scientific research:
Mechanism of Action
The mechanism by which 2,4-dinitrobenzoic acid exerts its effects is primarily through its reactive nitro groups. These groups can participate in various chemical reactions, making the compound a versatile intermediate in organic synthesis. The nitro groups also influence the compound’s reactivity towards nucleophiles and reducing agents, facilitating the formation of a wide range of derivatives.
Comparison with Similar Compounds
Similar compounds to 2,4-dinitrobenzoic acid include:
3,5-Dinitrobenzoic acid: This compound has nitro groups at the 3 and 5 positions of the benzene ring.
4-Nitrobenzoic acid: With a single nitro group at the 4 position, this compound is less reactive than this compound but is still used in various chemical syntheses.
2,4-Dinitrophenylhydrazine: This compound is used in the identification of carbonyl compounds and has similar reactivity due to the presence of nitro groups.
The uniqueness of this compound lies in its dual nitro substitution, which enhances its reactivity and makes it a valuable intermediate in organic synthesis.
Properties
IUPAC Name |
2,4-dinitrobenzoic acid | |
---|---|---|
Source | PubChem | |
URL | https://pubchem.ncbi.nlm.nih.gov | |
Description | Data deposited in or computed by PubChem | |
InChI |
InChI=1S/C7H4N2O6/c10-7(11)5-2-1-4(8(12)13)3-6(5)9(14)15/h1-3H,(H,10,11) | |
Source | PubChem | |
URL | https://pubchem.ncbi.nlm.nih.gov | |
Description | Data deposited in or computed by PubChem | |
InChI Key |
ZIIGSRYPZWDGBT-UHFFFAOYSA-N | |
Source | PubChem | |
URL | https://pubchem.ncbi.nlm.nih.gov | |
Description | Data deposited in or computed by PubChem | |
Canonical SMILES |
C1=CC(=C(C=C1[N+](=O)[O-])[N+](=O)[O-])C(=O)O | |
Source | PubChem | |
URL | https://pubchem.ncbi.nlm.nih.gov | |
Description | Data deposited in or computed by PubChem | |
Molecular Formula |
C7H4N2O6 | |
Source | PubChem | |
URL | https://pubchem.ncbi.nlm.nih.gov | |
Description | Data deposited in or computed by PubChem | |
DSSTOX Substance ID |
DTXSID3060584 | |
Record name | Benzoic acid, 2,4-dinitro- | |
Source | EPA DSSTox | |
URL | https://comptox.epa.gov/dashboard/DTXSID3060584 | |
Description | DSSTox provides a high quality public chemistry resource for supporting improved predictive toxicology. | |
Molecular Weight |
212.12 g/mol | |
Source | PubChem | |
URL | https://pubchem.ncbi.nlm.nih.gov | |
Description | Data deposited in or computed by PubChem | |
CAS No. |
610-30-0 | |
Record name | 2,4-Dinitrobenzoic acid | |
Source | CAS Common Chemistry | |
URL | https://commonchemistry.cas.org/detail?cas_rn=610-30-0 | |
Description | CAS Common Chemistry is an open community resource for accessing chemical information. Nearly 500,000 chemical substances from CAS REGISTRY cover areas of community interest, including common and frequently regulated chemicals, and those relevant to high school and undergraduate chemistry classes. This chemical information, curated by our expert scientists, is provided in alignment with our mission as a division of the American Chemical Society. | |
Explanation | The data from CAS Common Chemistry is provided under a CC-BY-NC 4.0 license, unless otherwise stated. | |
Record name | 2,4-Dinitrobenzoic acid | |
Source | ChemIDplus | |
URL | https://pubchem.ncbi.nlm.nih.gov/substance/?source=chemidplus&sourceid=0000610300 | |
Description | ChemIDplus is a free, web search system that provides access to the structure and nomenclature authority files used for the identification of chemical substances cited in National Library of Medicine (NLM) databases, including the TOXNET system. | |
Record name | 2,4-DINITROBENZOIC ACID | |
Source | DTP/NCI | |
URL | https://dtp.cancer.gov/dtpstandard/servlet/dwindex?searchtype=NSC&outputformat=html&searchlist=243713 | |
Description | The NCI Development Therapeutics Program (DTP) provides services and resources to the academic and private-sector research communities worldwide to facilitate the discovery and development of new cancer therapeutic agents. | |
Explanation | Unless otherwise indicated, all text within NCI products is free of copyright and may be reused without our permission. Credit the National Cancer Institute as the source. | |
Record name | Benzoic acid, 2,4-dinitro- | |
Source | EPA Chemicals under the TSCA | |
URL | https://www.epa.gov/chemicals-under-tsca | |
Description | EPA Chemicals under the Toxic Substances Control Act (TSCA) collection contains information on chemicals and their regulations under TSCA, including non-confidential content from the TSCA Chemical Substance Inventory and Chemical Data Reporting. | |
Record name | Benzoic acid, 2,4-dinitro- | |
Source | EPA DSSTox | |
URL | https://comptox.epa.gov/dashboard/DTXSID3060584 | |
Description | DSSTox provides a high quality public chemistry resource for supporting improved predictive toxicology. | |
Record name | 2,4-dinitrobenzoic acid | |
Source | European Chemicals Agency (ECHA) | |
URL | https://echa.europa.eu/substance-information/-/substanceinfo/100.009.291 | |
Description | The European Chemicals Agency (ECHA) is an agency of the European Union which is the driving force among regulatory authorities in implementing the EU's groundbreaking chemicals legislation for the benefit of human health and the environment as well as for innovation and competitiveness. | |
Explanation | Use of the information, documents and data from the ECHA website is subject to the terms and conditions of this Legal Notice, and subject to other binding limitations provided for under applicable law, the information, documents and data made available on the ECHA website may be reproduced, distributed and/or used, totally or in part, for non-commercial purposes provided that ECHA is acknowledged as the source: "Source: European Chemicals Agency, http://echa.europa.eu/". Such acknowledgement must be included in each copy of the material. ECHA permits and encourages organisations and individuals to create links to the ECHA website under the following cumulative conditions: Links can only be made to webpages that provide a link to the Legal Notice page. | |
Record name | 2,4-Dinitrobenzoic acid | |
Source | FDA Global Substance Registration System (GSRS) | |
URL | https://gsrs.ncats.nih.gov/ginas/app/beta/substances/L3FBD88RA8 | |
Description | The FDA Global Substance Registration System (GSRS) enables the efficient and accurate exchange of information on what substances are in regulated products. Instead of relying on names, which vary across regulatory domains, countries, and regions, the GSRS knowledge base makes it possible for substances to be defined by standardized, scientific descriptions. | |
Explanation | Unless otherwise noted, the contents of the FDA website (www.fda.gov), both text and graphics, are not copyrighted. They are in the public domain and may be republished, reprinted and otherwise used freely by anyone without the need to obtain permission from FDA. Credit to the U.S. Food and Drug Administration as the source is appreciated but not required. | |
Retrosynthesis Analysis
AI-Powered Synthesis Planning: Our tool employs the Template_relevance Pistachio, Template_relevance Bkms_metabolic, Template_relevance Pistachio_ringbreaker, Template_relevance Reaxys, Template_relevance Reaxys_biocatalysis model, leveraging a vast database of chemical reactions to predict feasible synthetic routes.
One-Step Synthesis Focus: Specifically designed for one-step synthesis, it provides concise and direct routes for your target compounds, streamlining the synthesis process.
Accurate Predictions: Utilizing the extensive PISTACHIO, BKMS_METABOLIC, PISTACHIO_RINGBREAKER, REAXYS, REAXYS_BIOCATALYSIS database, our tool offers high-accuracy predictions, reflecting the latest in chemical research and data.
Strategy Settings
Precursor scoring | Relevance Heuristic |
---|---|
Min. plausibility | 0.01 |
Model | Template_relevance |
Template Set | Pistachio/Bkms_metabolic/Pistachio_ringbreaker/Reaxys/Reaxys_biocatalysis |
Top-N result to add to graph | 6 |
Feasible Synthetic Routes
Disclaimer and Information on In-Vitro Research Products
Please be aware that all articles and product information presented on BenchChem are intended solely for informational purposes. The products available for purchase on BenchChem are specifically designed for in-vitro studies, which are conducted outside of living organisms. In-vitro studies, derived from the Latin term "in glass," involve experiments performed in controlled laboratory settings using cells or tissues. It is important to note that these products are not categorized as medicines or drugs, and they have not received approval from the FDA for the prevention, treatment, or cure of any medical condition, ailment, or disease. We must emphasize that any form of bodily introduction of these products into humans or animals is strictly prohibited by law. It is essential to adhere to these guidelines to ensure compliance with legal and ethical standards in research and experimentation.