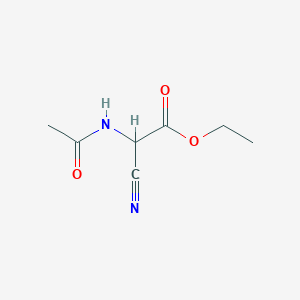
Ethyl acetamidocyanoacetate
Overview
Description
Ethyl acetamidocyanoacetate is an organic compound with the molecular formula C₇H₁₀N₂O₃ and a molecular weight of 170.17 g/mol . It is also known by other names such as ethyl 2-acetamido-2-cyanoacetate and glycine, N-acetyl-2-cyano-, ethyl ester . This compound is characterized by its white to off-white powder form and is primarily used in peptide synthesis .
Mechanism of Action
Ethyl acetamidocyanoacetate is a chemical compound with the linear formula CH3CONHCH(CN)CO2C2H5 . It has a molecular weight of 170.17 . This compound is used in various biochemical research, including proteomics . Here is a general overview based on the typical steps involved in the mechanism of action for similar compounds.
Preparation Methods
Synthetic Routes and Reaction Conditions: Ethyl acetamidocyanoacetate can be synthesized through the reaction of ethyl cyanoacetate with acetic anhydride in the presence of a base such as sodium acetate. The reaction typically proceeds under reflux conditions, resulting in the formation of this compound .
Industrial Production Methods: In industrial settings, the production of this compound involves similar synthetic routes but on a larger scale. The reaction conditions are optimized to ensure high yield and purity of the product. The compound is then purified through recrystallization or other suitable purification techniques .
Chemical Reactions Analysis
Types of Reactions: Ethyl acetamidocyanoacetate undergoes various chemical reactions, including:
Substitution Reactions: The compound can participate in nucleophilic substitution reactions due to the presence of the cyano and ester groups.
Common Reagents and Conditions:
Nucleophilic Substitution: Common reagents include nucleophiles such as amines or thiols.
Hydrolysis: Acidic or basic conditions using reagents like hydrochloric acid or sodium hydroxide.
Major Products Formed:
Substitution Reactions: Products include substituted derivatives of this compound.
Hydrolysis: Products include acetic acid, cyanoacetic acid, and the corresponding amine.
Scientific Research Applications
Ethyl acetamidocyanoacetate has a wide range of applications in scientific research:
Comparison with Similar Compounds
Ethyl acetamidocyanoacetate can be compared with other similar compounds such as:
Ethyl cyanoacetate: Similar in structure but lacks the acetamido group.
Ethyl 2-cyano-3-phenylacrylate: Contains a phenyl group instead of the acetamido group.
Ethyl 2-cyano-3-methylacrylate: Contains a methyl group instead of the acetamido group.
Properties
IUPAC Name |
ethyl 2-acetamido-2-cyanoacetate | |
---|---|---|
Source | PubChem | |
URL | https://pubchem.ncbi.nlm.nih.gov | |
Description | Data deposited in or computed by PubChem | |
InChI |
InChI=1S/C7H10N2O3/c1-3-12-7(11)6(4-8)9-5(2)10/h6H,3H2,1-2H3,(H,9,10) | |
Source | PubChem | |
URL | https://pubchem.ncbi.nlm.nih.gov | |
Description | Data deposited in or computed by PubChem | |
InChI Key |
SLIRLABNGAZSHX-UHFFFAOYSA-N | |
Source | PubChem | |
URL | https://pubchem.ncbi.nlm.nih.gov | |
Description | Data deposited in or computed by PubChem | |
Canonical SMILES |
CCOC(=O)C(C#N)NC(=O)C | |
Source | PubChem | |
URL | https://pubchem.ncbi.nlm.nih.gov | |
Description | Data deposited in or computed by PubChem | |
Molecular Formula |
C7H10N2O3 | |
Source | PubChem | |
URL | https://pubchem.ncbi.nlm.nih.gov | |
Description | Data deposited in or computed by PubChem | |
DSSTOX Substance ID |
DTXSID501292093 | |
Record name | Ethyl 2-(acetylamino)-2-cyanoacetate | |
Source | EPA DSSTox | |
URL | https://comptox.epa.gov/dashboard/DTXSID501292093 | |
Description | DSSTox provides a high quality public chemistry resource for supporting improved predictive toxicology. | |
Molecular Weight |
170.17 g/mol | |
Source | PubChem | |
URL | https://pubchem.ncbi.nlm.nih.gov | |
Description | Data deposited in or computed by PubChem | |
CAS No. |
4977-62-2 | |
Record name | Ethyl 2-(acetylamino)-2-cyanoacetate | |
Source | CAS Common Chemistry | |
URL | https://commonchemistry.cas.org/detail?cas_rn=4977-62-2 | |
Description | CAS Common Chemistry is an open community resource for accessing chemical information. Nearly 500,000 chemical substances from CAS REGISTRY cover areas of community interest, including common and frequently regulated chemicals, and those relevant to high school and undergraduate chemistry classes. This chemical information, curated by our expert scientists, is provided in alignment with our mission as a division of the American Chemical Society. | |
Explanation | The data from CAS Common Chemistry is provided under a CC-BY-NC 4.0 license, unless otherwise stated. | |
Record name | Ethyl acetamidocyanoacetate | |
Source | ChemIDplus | |
URL | https://pubchem.ncbi.nlm.nih.gov/substance/?source=chemidplus&sourceid=0004977622 | |
Description | ChemIDplus is a free, web search system that provides access to the structure and nomenclature authority files used for the identification of chemical substances cited in National Library of Medicine (NLM) databases, including the TOXNET system. | |
Record name | Ethyl acetamidocyanoacetate | |
Source | DTP/NCI | |
URL | https://dtp.cancer.gov/dtpstandard/servlet/dwindex?searchtype=NSC&outputformat=html&searchlist=49313 | |
Description | The NCI Development Therapeutics Program (DTP) provides services and resources to the academic and private-sector research communities worldwide to facilitate the discovery and development of new cancer therapeutic agents. | |
Explanation | Unless otherwise indicated, all text within NCI products is free of copyright and may be reused without our permission. Credit the National Cancer Institute as the source. | |
Record name | Ethyl acetamidocyanoacetate | |
Source | DTP/NCI | |
URL | https://dtp.cancer.gov/dtpstandard/servlet/dwindex?searchtype=NSC&outputformat=html&searchlist=8691 | |
Description | The NCI Development Therapeutics Program (DTP) provides services and resources to the academic and private-sector research communities worldwide to facilitate the discovery and development of new cancer therapeutic agents. | |
Explanation | Unless otherwise indicated, all text within NCI products is free of copyright and may be reused without our permission. Credit the National Cancer Institute as the source. | |
Record name | Ethyl 2-(acetylamino)-2-cyanoacetate | |
Source | EPA DSSTox | |
URL | https://comptox.epa.gov/dashboard/DTXSID501292093 | |
Description | DSSTox provides a high quality public chemistry resource for supporting improved predictive toxicology. | |
Record name | Ethyl acetamidocyanoacetate | |
Source | European Chemicals Agency (ECHA) | |
URL | https://echa.europa.eu/substance-information/-/substanceinfo/100.023.295 | |
Description | The European Chemicals Agency (ECHA) is an agency of the European Union which is the driving force among regulatory authorities in implementing the EU's groundbreaking chemicals legislation for the benefit of human health and the environment as well as for innovation and competitiveness. | |
Explanation | Use of the information, documents and data from the ECHA website is subject to the terms and conditions of this Legal Notice, and subject to other binding limitations provided for under applicable law, the information, documents and data made available on the ECHA website may be reproduced, distributed and/or used, totally or in part, for non-commercial purposes provided that ECHA is acknowledged as the source: "Source: European Chemicals Agency, http://echa.europa.eu/". Such acknowledgement must be included in each copy of the material. ECHA permits and encourages organisations and individuals to create links to the ECHA website under the following cumulative conditions: Links can only be made to webpages that provide a link to the Legal Notice page. | |
Retrosynthesis Analysis
AI-Powered Synthesis Planning: Our tool employs the Template_relevance Pistachio, Template_relevance Bkms_metabolic, Template_relevance Pistachio_ringbreaker, Template_relevance Reaxys, Template_relevance Reaxys_biocatalysis model, leveraging a vast database of chemical reactions to predict feasible synthetic routes.
One-Step Synthesis Focus: Specifically designed for one-step synthesis, it provides concise and direct routes for your target compounds, streamlining the synthesis process.
Accurate Predictions: Utilizing the extensive PISTACHIO, BKMS_METABOLIC, PISTACHIO_RINGBREAKER, REAXYS, REAXYS_BIOCATALYSIS database, our tool offers high-accuracy predictions, reflecting the latest in chemical research and data.
Strategy Settings
Precursor scoring | Relevance Heuristic |
---|---|
Min. plausibility | 0.01 |
Model | Template_relevance |
Template Set | Pistachio/Bkms_metabolic/Pistachio_ringbreaker/Reaxys/Reaxys_biocatalysis |
Top-N result to add to graph | 6 |
Feasible Synthetic Routes
A: Ethyl acetamidocyanoacetate serves as a crucial building block for various heterocycles. For instance, it undergoes Michael addition with α,β-unsaturated ketones, followed by FeCl3-mediated intramolecular ketone-nitrile annulation and aromatization, yielding 3-acetamido-2-pyridone derivatives. [] This reaction sequence highlights the reagent's ability to contribute to both the carbon framework and nitrogen atom of the pyridone ring.
A: this compound plays a pivotal role in a novel three-step synthesis of 3-aryl β-carbolin-1-ones. [, ] The process involves Michael addition of the reagent to chalcones, followed by an acid-mediated cyclization to form the pyridone ring. A subsequent Cu(I)-catalyzed intramolecular N-arylation furnishes the β-carbolin-1-one scaffold. These compounds exhibit significant in vitro activity against tumor cell proliferation, showcasing the potential of this compound in medicinal chemistry. []
A: this compound serves as a key starting material in synthesizing DL-ornithine. [] The process involves condensing the reagent with 3-bromopropylphthalimide, followed by hydrolysis of the resulting nitrile intermediate. This method provides a convenient route to access this essential amino acid.
A: this compound exhibits versatility in synthesizing various other amino acids. For example, it's used to prepare multilabeled histidine, specifically DL-histidine-α-13C-3-15N-2,5-2H2. [] This labeled histidine serves as a valuable tool in mass spectrometry for studying histidine metabolism. Furthermore, the reagent acts as a precursor in the synthesis of DL-lysine and DL-tyrosine labeled with isotopic carbon-14, providing valuable tools for metabolic studies. []
A: Fourteen structural analogues of ornithine, synthesized using this compound as a starting point, were evaluated as inhibitors of the enzyme L-ornithine carboxylase (ODC). [] This study aimed to understand the structural requirements for binding to the enzyme's active site. The findings revealed that (+)-α-methylornithine, synthesized from this compound, acted as a potent reversible inhibitor of ODC, providing valuable insights into the enzyme's mechanism.
A: The presence of the acidic methylene group, flanked by the acetamido and cyano groups, renders this compound susceptible to deprotonation, generating a stabilized carbanion. [] This carbanion readily undergoes alkylation reactions with various electrophiles. For example, in the synthesis of α-substituted serines as potential inhibitors of serine hydroxymethyltransferase, the anion derived from this compound was alkylated with appropriate reagents. [] This demonstrates the utility of the reagent in creating modified amino acid derivatives.
A: this compound, containing both amide (-NH) and ester (-COOR) groups, was employed as a model compound to investigate the micro-solvation of these functional groups in neat DMSO using 1H-NMR spectroscopy and computational methods. [] Results revealed that DMSO molecules engage in multiple strong interactions with the studied molecules, particularly with the carbonyl groups. These findings offer significant insights into DMSO's impact on biomolecular structures and its interactions with biomolecular functional groups.
A: p-(4-Hydroxybenzoyl)phenylalanine is a photoreactive amino acid analog with applications in studying peptide-protein interactions. [] Its synthesis involves a multistep procedure where this compound reacts with a benzophenone derivative. This reaction highlights the reagent's versatility in constructing complex amino acid analogs. The resulting p-(4-hydroxybenzoyl)phenylalanine can be radioiodinated, enabling the investigation of peptide interactions with their receptors, as demonstrated with the substance P receptor.
A: Triphenylphosphine catalyzes the reaction between this compound and alkyl propiolates, leading to polyfunctionalized alkenes and α,β-unsaturated γ-lactams. [, ] While the exact mechanism requires further investigation, it likely involves the formation of a phosphonium ylide intermediate. This ylide can then react with the alkyl propiolate through a Michael addition-cyclization cascade to afford the observed products. This reaction highlights the utility of this compound in forming complex molecular architectures.
Disclaimer and Information on In-Vitro Research Products
Please be aware that all articles and product information presented on BenchChem are intended solely for informational purposes. The products available for purchase on BenchChem are specifically designed for in-vitro studies, which are conducted outside of living organisms. In-vitro studies, derived from the Latin term "in glass," involve experiments performed in controlled laboratory settings using cells or tissues. It is important to note that these products are not categorized as medicines or drugs, and they have not received approval from the FDA for the prevention, treatment, or cure of any medical condition, ailment, or disease. We must emphasize that any form of bodily introduction of these products into humans or animals is strictly prohibited by law. It is essential to adhere to these guidelines to ensure compliance with legal and ethical standards in research and experimentation.