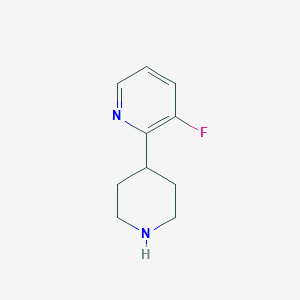
3-Fluoro-2-(piperidin-4-yl)pyridine
Overview
Description
3-Fluoro-2-(piperidin-4-yl)pyridine is a heterocyclic compound featuring a pyridine ring substituted with a fluorine atom at position 3 and a piperidine moiety at position 2. The fluorine atom at position 3 enhances electronegativity and may influence metabolic stability, lipophilicity, and bioavailability.
Preparation Methods
Preparation Methods of 3-Fluoro-2-(piperidin-4-yl)pyridine
Fluorination of Pyridine Derivatives
A common strategy to prepare fluoropyridine intermediates involves selective fluorination of pyridine rings, which can later be converted to the target compound. One patented method begins with quinolinic acid, which is converted through several steps into 3-fluoro-2-pyridinecarboxylic acid isopropyl ester, a key fluorinated intermediate. The steps include:
- Conversion of quinolinic acid to quinoline anhydride using thionyl chloride.
- Esterification with isopropanol to obtain 2,3-pyridinedioic acid-2-isopropyl ester.
- Reaction with diphenylphosphoryl azide (DPPA) and triethylamine to form an intermediate amine ester.
- Fluorination using pyridine hydrogen fluoride and sodium nitrite at controlled low temperatures.
- Reduction of the fluorinated ester with sodium borohydride and calcium chloride to yield 3-fluoropyridine-2-methanol.
This sequence highlights the use of mild fluorination conditions and controlled temperature to achieve selective fluorination at the 3-position of the pyridine ring.
Catalytic Hydrogenation and Dearomatization
Another approach involves the catalytic hydrogenation of fluoropyridine derivatives to form fluorinated piperidine rings directly. Using rhodium-based catalysts (e.g., Rh-CAAC), 3-fluoropyridine substrates undergo selective dearomatization and hydrogenation to produce fluorinated piperidine intermediates with high chemo- and diastereoselectivity. This method allows the synthesis of single isomers, which is crucial for biological activity:
- The process uses a pyridine reducing system with H-Bpin and rhodium catalysts.
- Hydrogenation proceeds with high diastereoselectivity, favoring the cis fluorinated piperidine isomer.
- Isolation often involves derivatization to trifluoroacetamides to stabilize volatile products.
- Enzyme-catalyzed asymmetric transamination has also been explored to introduce stereochemistry, starting from fluoroketone intermediates prepared via electrophilic fluorination (e.g., with Selectfluor).
This method is notable for combining catalytic hydrogenation with enzymatic steps to achieve stereoselective synthesis of fluorinated piperidines.
Photoredox-Mediated Coupling for Fluoropyridines
Photoredox catalysis has been employed to synthesize 3-fluoropyridines from ketone precursors via silyl enol ether intermediates:
- Silyl enol ethers are prepared from ketones using chlorotrimethylsilane and triethylamine in acetonitrile under inert atmosphere.
- These intermediates undergo photoredox-mediated coupling to form 3-fluoropyridines.
- This method offers versatility in substituent patterns on the pyridine ring but requires careful control of reaction conditions.
Though this method focuses on fluoropyridines, it provides a platform for further functionalization to piperidinyl derivatives.
Palladium and Rhodium-Catalyzed Hydrogenation and Coupling
Recent advances include palladium- and rhodium-catalyzed one-pot hydrogenation and coupling reactions to form substituted piperidines from pyridine precursors:
- Grygorenko et al. demonstrated a method combining metalation removal, dehydroxylation, and pyridine reduction in one step.
- Rhodium catalysts are particularly effective for 3-substituted piperidines bearing fluorinated groups, operating under mild conditions with shorter reaction times.
- The process can be tuned to retain hydroxyl groups or achieve full reduction depending on additives (e.g., triethylamine vs. hydrochloric acid).
- Suzuki–Miyaura coupling followed by hydrogenation enables the synthesis of functionalized piperidines with high chemoselectivity and yield.
This approach is valuable for preparing this compound derivatives with diverse substituents.
Nucleophilic Aromatic Substitution and Coupling Strategies
In medicinal chemistry applications, nucleophilic substitution of fluorine in 3-fluoropyridines is used to introduce piperidinyl groups:
- Replacement of the fluoro group in 3-fluoropyridine derivatives by piperidine-based nucleophiles under acidic or high-temperature conditions.
- Pd-catalyzed C–N bond formation (Buchwald-Hartwig amination) using 3-bromo-4-chloropyridine and piperidine derivatives.
- Subsequent functional group transformations (e.g., hydrolysis, condensation) yield the target 3-piperidinyl pyridine compounds.
- These methods enable selective substitution at the 3-position and installation of piperidin-4-yl substituents with control over regio- and stereochemistry.
Comparative Data Table of Preparation Methods
Research Findings and Notes
- The fluorination step often dictates the regioselectivity and yield of the final product. Use of pyridine hydrogen fluoride and sodium nitrite at low temperatures is effective for selective 3-fluorination.
- Rhodium catalysts are superior to palladium in hydrogenation of fluoropyridines to piperidines, providing better stereoselectivity and milder conditions.
- Enzymatic transamination offers a promising route to stereoselective synthesis but requires optimization to avoid defluorination side reactions.
- Photoredox methods expand the toolbox for fluoropyridine synthesis but are less directly applied to piperidinyl derivatives without further steps.
- Nucleophilic aromatic substitution and Pd-catalyzed amination are widely used in medicinal chemistry for late-stage functionalization, enabling diverse piperidinyl derivatives.
Chemical Reactions Analysis
Types of Reactions
3-Fluoro-2-(piperidin-4-yl)pyridine can undergo various types of chemical reactions, including:
Oxidation: The compound can be oxidized to form N-oxides.
Reduction: Reduction reactions can convert the compound into different amines.
Substitution: Nucleophilic substitution reactions are common, where the fluorine atom can be replaced by other nucleophiles.
Common Reagents and Conditions
Oxidation: Common oxidizing agents include hydrogen peroxide and m-chloroperbenzoic acid.
Reduction: Reducing agents such as lithium aluminum hydride (LiAlH4) are often used.
Substitution: Reagents like potassium fluoride (KF) and sodium hydride (NaH) are used in substitution reactions.
Major Products
The major products formed from these reactions depend on the specific reagents and conditions used. For example, oxidation can yield N-oxides, while substitution reactions can produce various fluorinated derivatives .
Scientific Research Applications
Therapeutic Potential
The compound is being investigated for its potential in treating various diseases, including:
- Cancer : Research indicates that derivatives of compounds similar to 3-Fluoro-2-(piperidin-4-yl)pyridine exhibit anticancer properties. For instance, studies have shown that certain thiadiazole derivatives can decrease the viability of various cancer cell lines, suggesting a role for this compound in cancer treatment .
- Neurological Disorders : Given its structural similarity to known neuroactive compounds, this compound may also be explored for its effects on neurodegenerative diseases such as Alzheimer's and Parkinson's disease. Its interaction with specific molecular targets could lead to modulation of pathways involved in these disorders .
Biological Studies
In biological research, this compound is utilized to explore its interactions with various biological targets:
- Target Interaction Studies : Understanding how this compound interacts with enzymes or receptors can provide insights into its mechanism of action. Such studies are essential for elucidating how it may inhibit or activate specific biological pathways .
Pharmacological Research
Pharmacological studies focus on several key aspects of this compound:
- Pharmacokinetics and Bioavailability : Investigations into how the compound is absorbed, distributed, metabolized, and excreted can help determine its efficacy as a therapeutic agent. Ongoing studies evaluate its bioavailability and toxicity profiles .
Industrial Applications
In addition to medicinal applications, this compound may serve as an intermediate in the synthesis of more complex pharmaceutical molecules. This aspect highlights its utility in drug development processes where multi-step synthesis is often required .
Case Studies and Research Findings
Several studies have documented the biological activity of compounds similar to this compound:
Study | Focus Area | Findings |
---|---|---|
Study A | Anticancer Activity | Demonstrated that derivatives reduced viability in cancer cell lines significantly . |
Study B | Neuroactive Properties | Identified potential modulation of pathways related to Alzheimer's disease through receptor interaction . |
Study C | Pharmacokinetics | Provided data on absorption and distribution profiles indicating favorable bioavailability . |
Mechanism of Action
The mechanism of action of 3-Fluoro-2-(piperidin-4-yl)pyridine involves its interaction with specific molecular targets and pathways. The fluorine atom in the pyridine ring enhances the compound’s ability to interact with biological targets, potentially leading to the inhibition of enzymes or receptors involved in disease processes . The exact molecular targets and pathways can vary depending on the specific application and context of use.
Comparison with Similar Compounds
Comparison with Structurally Similar Compounds
Halogen-Substituted Pyridine Derivatives
Compounds with halogen substituents on pyridine, such as chlorine or fluorine, are common in drug discovery due to their electronic and steric effects.
Key Observations :
- Chlorine substituents (e.g., in ) correlate with higher melting points (~268–287°C), suggesting enhanced crystallinity and stability compared to fluorine analogs.
Piperidine-Containing Pyridine Compounds
Piperidine moieties are prevalent in CNS-active drugs due to their ability to cross the blood-brain barrier (BBB).
Key Observations :
- The donepezil hybrid in demonstrates that piperidine-pyridine hybrids can achieve potent AChE inhibition (nanomolar IC₅₀) and Aβ aggregation suppression. The methylpyridine group enhances BBB penetration.
- Trifluoromethyl groups (e.g., in ) increase lipophilicity and electron-withdrawing effects, critical for JAK inhibitor potency. The absence of a trifluoromethyl group in this compound may reduce kinase affinity but improve solubility.
Fluorinated Pyridine-Based Pharmacophores
Fluorine is often used to optimize pharmacokinetic properties.
Key Observations :
- Trifluoromethyl groups (e.g., in ) enhance JAK binding via hydrophobic interactions, but their absence in this compound may limit this effect.
Biological Activity
3-Fluoro-2-(piperidin-4-yl)pyridine is a heterocyclic compound that has garnered attention for its potential biological activities, particularly in the fields of pharmacology and medicinal chemistry. This article explores the compound's biological activity, mechanisms of action, and its comparative analysis with similar compounds.
Chemical Structure and Properties
The molecular structure of this compound comprises a fluorinated pyridine ring and a piperidine moiety. The presence of the fluorine atom enhances the compound's lipophilicity and metabolic stability, making it a promising candidate for various pharmaceutical applications. Its molecular formula is CHFN.
The biological activity of this compound is primarily attributed to its interaction with specific molecular targets. The fluorine substitution in the pyridine ring increases the compound's ability to engage with biological receptors, potentially inhibiting enzymes or modulating receptor activity involved in disease processes.
Key Mechanisms:
- Enzyme Inhibition : The compound may inhibit key enzymes related to metabolic disorders and cancer pathways.
- Receptor Modulation : It acts as a modulator for neurotransmitter receptors in the central nervous system, which can influence pain pathways and other neurological functions.
Biological Activities
Research has highlighted several biological activities associated with this compound:
- Antimicrobial Activity : Preliminary studies suggest potential antimicrobial properties against various pathogens.
- Anticancer Properties : The compound has been explored for its anticancer effects, particularly in inhibiting tumor cell proliferation.
- Analgesic Effects : It may exhibit analgesic properties by modulating pain pathways, as evidenced by studies on related compounds .
Comparative Analysis with Similar Compounds
To understand the uniqueness of this compound, it is essential to compare it with structurally similar compounds:
Compound Name | Structure Features | Biological Activity |
---|---|---|
3-Fluoropyridine | Simpler fluorinated pyridine | Antimicrobial properties |
Piperidine | Basic nitrogen-containing heterocycle | Foundational structure in pharmaceuticals |
3-Fluoro-N-(piperidin-4-yl)aniline | Aniline derivative; amine functional group | Inhibitory effects on protein kinases |
3-Fluoro-4-(morpholinyl)pyridine | Morpholine ring substitution | Antimicrobial activity |
This table illustrates how slight modifications can lead to significant variations in biological potency and selectivity.
Case Studies and Research Findings
Several case studies have investigated the biological activity of compounds related to this compound:
- TRPV1 Antagonism : A study demonstrated that derivatives similar to this compound exhibited potent antagonism towards TRPV1 receptors, which are implicated in pain signaling pathways. These compounds showed significant anti-allodynic effects in neuropathic pain models .
- Antiparasitic Activity : Research on pyridyl derivatives indicated varying degrees of antiparasitic activity, suggesting that modifications in the piperidine and pyridine rings can enhance efficacy against specific targets .
- Neuroactive Properties : Investigations into neuroactive properties revealed that compounds with similar structures could modulate neurotransmitter systems effectively, indicating potential therapeutic applications in treating neurological disorders.
Q & A
Basic Questions
Q. What are the standard synthetic routes for 3-Fluoro-2-(piperidin-4-yl)pyridine?
- Methodology : The compound is typically synthesized via nucleophilic substitution or coupling reactions. For example, intermediates like tert-butyl carbamate derivatives (e.g., tert-butyl (1-(2-chloronicotinoyl)piperidin-4-yl)carbamate) can undergo fluorination using potassium fluoride or similar reagents under controlled conditions. Reaction steps often involve dichloromethane as a solvent and sodium hydroxide for neutralization, followed by purification via column chromatography .
Q. Which spectroscopic techniques are recommended for characterizing this compound?
- Methodology :
- NMR : 1H, 13C, and 19F NMR to confirm fluorine substitution and piperidine ring conformation.
- HPLC/MS : For purity assessment and molecular weight verification.
- X-ray crystallography : Tools like Mercury CSD 2.0 () enable structural validation by comparing experimental data with crystallographic databases .
Q. What are the primary pharmacological applications of this compound?
- Methodology : The compound serves as a precursor in Janus kinase (JAK) inhibitors () and antihistamines (e.g., desloratadine impurities in ). Biological activity is assessed via in vitro kinase assays or receptor-binding studies using fluorescence polarization or surface plasmon resonance (SPR) .
Advanced Research Questions
Q. How can reaction conditions be optimized to minimize by-products during synthesis?
- Methodology :
- Stoichiometric control : Limit excess reagents to reduce side reactions (e.g., over-fluorination).
- Temperature modulation : Lower temperatures mitigate thermal degradation.
- Catalyst screening : Palladium-based catalysts improve coupling efficiency.
- Purification : Use preparative HPLC or recrystallization (as in , achieving 99% purity) .
Q. How can contradictions in spectroscopic data (e.g., NMR splitting patterns) be resolved?
- Methodology :
- 19F NMR : Resolves ambiguities caused by fluorine’s magnetic anisotropy.
- 2D NMR techniques : HSQC or COSY correlations clarify proton-carbon/fluorine connectivity.
- Computational modeling : Density functional theory (DFT) predicts chemical shifts for comparison with experimental data .
Q. What strategies are effective for assessing binding affinity to target proteins (e.g., JAK enzymes)?
- Methodology :
- Isothermal titration calorimetry (ITC) : Quantifies thermodynamic binding parameters.
- Molecular docking : Software like Mercury CSD 2.0 () models ligand-protein interactions using crystallographic data.
- Competitive binding assays : Fluorescent probes (e.g., TR-FRET) measure displacement efficiency .
Q. How can computational tools address challenges in structural elucidation?
- Methodology :
- Mercury CSD Materials Module : Identifies intermolecular interaction motifs in crystallographic data ().
- Conformational analysis : Molecular dynamics simulations predict stable conformers of the piperidine ring under physiological conditions .
Q. What are the critical considerations for scaling up synthesis from milligram to gram quantities?
- Methodology :
- Solvent selection : Replace dichloromethane with safer alternatives (e.g., ethyl acetate) for large-scale reactions.
- Safety protocols : Mitigate risks of exothermic reactions via controlled reagent addition (see safety guidelines in ).
- Process analytical technology (PAT) : Real-time monitoring via IR spectroscopy ensures reaction completion .
Q. Data Contradiction Analysis
Q. How to reconcile discrepancies between computational predictions and experimental crystallographic data?
- Methodology :
- Error analysis : Compare root-mean-square deviations (RMSD) between predicted and observed bond lengths/angles.
- Twinning refinement : Use SHELXL ( ) to model twinned crystals when diffraction patterns are ambiguous.
- Hirshfeld surface analysis : Visualizes intermolecular interactions to validate packing patterns .
Q. What steps validate the purity of this compound when HPLC and NMR results conflict?
- Methodology :
- Orthogonal techniques : Combine mass spectrometry (HRMS) and elemental analysis.
- Spiking experiments : Add a known impurity to confirm retention times.
- Thermogravimetric analysis (TGA) : Detects volatile by-products not visible via NMR .
Q. Tables for Key Data
Parameter | Technique | Reference |
---|---|---|
Fluorine substitution | 19F NMR | |
Crystal structure validation | Mercury CSD 2.0 | |
Purity assessment (>99%) | Preparative HPLC | |
Binding affinity measurement | ITC/SPR |
Properties
IUPAC Name |
3-fluoro-2-piperidin-4-ylpyridine | |
---|---|---|
Source | PubChem | |
URL | https://pubchem.ncbi.nlm.nih.gov | |
Description | Data deposited in or computed by PubChem | |
InChI |
InChI=1S/C10H13FN2/c11-9-2-1-5-13-10(9)8-3-6-12-7-4-8/h1-2,5,8,12H,3-4,6-7H2 | |
Source | PubChem | |
URL | https://pubchem.ncbi.nlm.nih.gov | |
Description | Data deposited in or computed by PubChem | |
InChI Key |
DLPZRBXJTPJPHJ-UHFFFAOYSA-N | |
Source | PubChem | |
URL | https://pubchem.ncbi.nlm.nih.gov | |
Description | Data deposited in or computed by PubChem | |
Canonical SMILES |
C1CNCCC1C2=C(C=CC=N2)F | |
Source | PubChem | |
URL | https://pubchem.ncbi.nlm.nih.gov | |
Description | Data deposited in or computed by PubChem | |
Molecular Formula |
C10H13FN2 | |
Source | PubChem | |
URL | https://pubchem.ncbi.nlm.nih.gov | |
Description | Data deposited in or computed by PubChem | |
Molecular Weight |
180.22 g/mol | |
Source | PubChem | |
URL | https://pubchem.ncbi.nlm.nih.gov | |
Description | Data deposited in or computed by PubChem | |
Retrosynthesis Analysis
AI-Powered Synthesis Planning: Our tool employs the Template_relevance Pistachio, Template_relevance Bkms_metabolic, Template_relevance Pistachio_ringbreaker, Template_relevance Reaxys, Template_relevance Reaxys_biocatalysis model, leveraging a vast database of chemical reactions to predict feasible synthetic routes.
One-Step Synthesis Focus: Specifically designed for one-step synthesis, it provides concise and direct routes for your target compounds, streamlining the synthesis process.
Accurate Predictions: Utilizing the extensive PISTACHIO, BKMS_METABOLIC, PISTACHIO_RINGBREAKER, REAXYS, REAXYS_BIOCATALYSIS database, our tool offers high-accuracy predictions, reflecting the latest in chemical research and data.
Strategy Settings
Precursor scoring | Relevance Heuristic |
---|---|
Min. plausibility | 0.01 |
Model | Template_relevance |
Template Set | Pistachio/Bkms_metabolic/Pistachio_ringbreaker/Reaxys/Reaxys_biocatalysis |
Top-N result to add to graph | 6 |
Feasible Synthetic Routes
Disclaimer and Information on In-Vitro Research Products
Please be aware that all articles and product information presented on BenchChem are intended solely for informational purposes. The products available for purchase on BenchChem are specifically designed for in-vitro studies, which are conducted outside of living organisms. In-vitro studies, derived from the Latin term "in glass," involve experiments performed in controlled laboratory settings using cells or tissues. It is important to note that these products are not categorized as medicines or drugs, and they have not received approval from the FDA for the prevention, treatment, or cure of any medical condition, ailment, or disease. We must emphasize that any form of bodily introduction of these products into humans or animals is strictly prohibited by law. It is essential to adhere to these guidelines to ensure compliance with legal and ethical standards in research and experimentation.