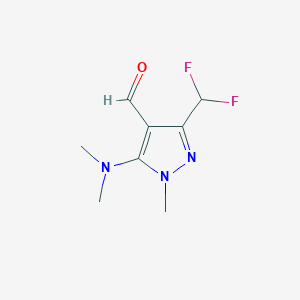
3-(Difluoromethyl)-5-(dimethylamino)-1-methyl-1H-pyrazole-4-carbaldehyde
Overview
Description
3-(Difluoromethyl)-5-(dimethylamino)-1-methyl-1H-pyrazole-4-carbaldehyde is a useful research compound. Its molecular formula is C8H11F2N3O and its molecular weight is 203.19 g/mol. The purity is usually 95%.
BenchChem offers high-quality this compound suitable for many research applications. Different packaging options are available to accommodate customers' requirements. Please inquire for more information about this compound including the price, delivery time, and more detailed information at info@benchchem.com.
Biological Activity
3-(Difluoromethyl)-5-(dimethylamino)-1-methyl-1H-pyrazole-4-carbaldehyde (CAS 877133-03-4) is a pyrazole derivative that has garnered attention for its potential biological activities, particularly in medicinal chemistry and agricultural applications. Pyrazole compounds are known for their diverse pharmacological properties, including anti-inflammatory, anticancer, and antifungal activities. This article reviews the biological activity of this specific compound, synthesizing findings from various studies to provide a comprehensive overview.
Chemical Structure and Properties
The molecular structure of this compound can be described as follows:
- IUPAC Name : 3-(difluoromethyl)-5-(dimethylamino)-1-methylpyrazole-4-carbaldehyde
- Molecular Formula : C8H11F2N3O
- Molecular Weight : 201.19 g/mol
Structural Representation
Property | Value |
---|---|
InChI Key | DNFWBOYYZVXTIC-UHFFFAOYSA-N |
SMILES | CN(C)C1=NN(C(=O)C=C1F)F |
Antifungal Activity
Recent studies have highlighted the antifungal potential of pyrazole derivatives, including this compound. A series of derivatives synthesized from this compound were tested against various phytopathogenic fungi. The results indicated that many exhibited moderate to excellent antifungal activity, with some derivatives outperforming standard antifungal agents like boscalid in in vitro mycelial growth inhibition assays .
Antitumor Activity
Pyrazole derivatives have also shown promise as antitumor agents. Research indicates that compounds in this class can inhibit key cancer-related pathways, including BRAF(V600E), which is significant in melanoma treatment. The structure-activity relationship (SAR) studies suggest that modifications to the pyrazole ring can enhance anticancer efficacy . Specific derivatives have been tested against breast cancer cell lines, demonstrating potential synergistic effects when combined with established chemotherapeutics like doxorubicin .
Anti-inflammatory Activity
The anti-inflammatory properties of pyrazoles are well-documented. Compounds similar to this compound have shown significant inhibition of pro-inflammatory cytokines such as TNF-α and IL-6 in vitro. This suggests potential therapeutic applications in treating inflammatory diseases .
Synthesis and Testing of Derivatives
A notable study synthesized a series of 3-(difluoromethyl)-1-methyl-1H-pyrazole-4-carboxylic acid amides and evaluated their biological activities against seven phytopathogenic fungi. Among these, one derivative exhibited superior antifungal activity compared to established fungicides, highlighting the compound's potential as an agrochemical .
Molecular Docking Studies
Molecular docking studies have provided insights into the interaction mechanisms of these compounds at the molecular level. For instance, one derivative was found to form hydrogen bonds with critical amino acids in target proteins involved in fungal growth, suggesting a specific mode of action that could be exploited for drug design .
Scientific Research Applications
Synthesis Methodology
- Starting Materials : The synthesis often begins with readily available pyrazole derivatives.
- Reaction Conditions : The reactions are usually conducted in organic solvents like dichloromethane or dioxane, employing catalysts such as sodium iodide or potassium iodide.
- Yield : Reports indicate that yields can vary but often exceed 50% depending on the specific reaction conditions used .
Antifungal Properties
Research has demonstrated that derivatives of 3-(difluoromethyl)-5-(dimethylamino)-1-methyl-1H-pyrazole-4-carbaldehyde exhibit notable antifungal activity. For instance, a series of amides derived from this compound were tested against various phytopathogenic fungi, showing superior activity compared to standard fungicides like boscalid .
- Mechanism of Action : The antifungal activity is believed to be linked to the compound's ability to inhibit specific enzymes involved in fungal metabolism, potentially disrupting cell wall synthesis or function.
Pesticidal Applications
The compound is also being explored for its utility in developing novel pesticides. Pyrazole derivatives are recognized for their effectiveness as agrochemicals due to their low toxicity and high efficiency .
- Case Studies : Several studies have highlighted the synthesis of new pyrazole-based fungicides that leverage the structural features of this compound to enhance efficacy against resistant strains of agricultural pests.
Comparison of Biological Activities
Properties
IUPAC Name |
3-(difluoromethyl)-5-(dimethylamino)-1-methylpyrazole-4-carbaldehyde | |
---|---|---|
Source | PubChem | |
URL | https://pubchem.ncbi.nlm.nih.gov | |
Description | Data deposited in or computed by PubChem | |
InChI |
InChI=1S/C8H11F2N3O/c1-12(2)8-5(4-14)6(7(9)10)11-13(8)3/h4,7H,1-3H3 | |
Source | PubChem | |
URL | https://pubchem.ncbi.nlm.nih.gov | |
Description | Data deposited in or computed by PubChem | |
InChI Key |
DNFWBOYYZVXTIC-UHFFFAOYSA-N | |
Source | PubChem | |
URL | https://pubchem.ncbi.nlm.nih.gov | |
Description | Data deposited in or computed by PubChem | |
Canonical SMILES |
CN1C(=C(C(=N1)C(F)F)C=O)N(C)C | |
Source | PubChem | |
URL | https://pubchem.ncbi.nlm.nih.gov | |
Description | Data deposited in or computed by PubChem | |
Molecular Formula |
C8H11F2N3O | |
Source | PubChem | |
URL | https://pubchem.ncbi.nlm.nih.gov | |
Description | Data deposited in or computed by PubChem | |
Molecular Weight |
203.19 g/mol | |
Source | PubChem | |
URL | https://pubchem.ncbi.nlm.nih.gov | |
Description | Data deposited in or computed by PubChem | |
Synthesis routes and methods
Procedure details
Retrosynthesis Analysis
AI-Powered Synthesis Planning: Our tool employs the Template_relevance Pistachio, Template_relevance Bkms_metabolic, Template_relevance Pistachio_ringbreaker, Template_relevance Reaxys, Template_relevance Reaxys_biocatalysis model, leveraging a vast database of chemical reactions to predict feasible synthetic routes.
One-Step Synthesis Focus: Specifically designed for one-step synthesis, it provides concise and direct routes for your target compounds, streamlining the synthesis process.
Accurate Predictions: Utilizing the extensive PISTACHIO, BKMS_METABOLIC, PISTACHIO_RINGBREAKER, REAXYS, REAXYS_BIOCATALYSIS database, our tool offers high-accuracy predictions, reflecting the latest in chemical research and data.
Strategy Settings
Precursor scoring | Relevance Heuristic |
---|---|
Min. plausibility | 0.01 |
Model | Template_relevance |
Template Set | Pistachio/Bkms_metabolic/Pistachio_ringbreaker/Reaxys/Reaxys_biocatalysis |
Top-N result to add to graph | 6 |
Feasible Synthetic Routes
Disclaimer and Information on In-Vitro Research Products
Please be aware that all articles and product information presented on BenchChem are intended solely for informational purposes. The products available for purchase on BenchChem are specifically designed for in-vitro studies, which are conducted outside of living organisms. In-vitro studies, derived from the Latin term "in glass," involve experiments performed in controlled laboratory settings using cells or tissues. It is important to note that these products are not categorized as medicines or drugs, and they have not received approval from the FDA for the prevention, treatment, or cure of any medical condition, ailment, or disease. We must emphasize that any form of bodily introduction of these products into humans or animals is strictly prohibited by law. It is essential to adhere to these guidelines to ensure compliance with legal and ethical standards in research and experimentation.