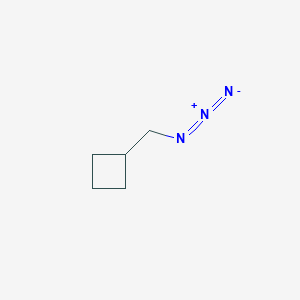
Azidomethylcyclobutane
Overview
Description
Azidomethylcyclobutane (AMCB) is an organic compound belonging to the class of cyclobutanes. It is a four-membered ring compound composed of four carbon atoms and one nitrogen atom. AMCB is a versatile and highly reactive compound, which has been widely used in various scientific research applications.
Scientific Research Applications
Bioactive Properties and Drug Discovery
Azidomethylcyclobutane and its derivatives have been extensively studied for their bioactive properties. Research highlights the significant antimicrobial, antibacterial, anticancer, and other bioactivities of cyclobutane-containing alkaloids, including synthetic compounds isolated from terrestrial and marine species. These compounds, including this compound, have shown promise in drug discovery, contributing to the progress of medicine by expanding the therapeutic armamentarium and enriching it with novel bioactive molecules. The synthesis, origins, and biological activities of cyclobutane-containing alkaloids, including this compound and its derivatives, underscore their potential in drug discovery and development (Dembitsky, 2007; Sergeiko et al., 2008).
Chemical and Biological Applications
This compound's unique structure and properties have made it a focal point in the field of chemical and biological research. The compound and its derivatives have been integral in the development of novel methodologies, such as strain-release heteroatom functionalization. This approach has been applied in various contexts, including bioconjugation and peptide labeling, highlighting the compound's versatility and its potential role in advancing chemical research and drug development (Lopchuk et al., 2017).
Mechanism of Action
Target of action
The target of a compound is usually a protein in the body with which the compound interacts. This could be an enzyme, a receptor, or any other protein that plays a role in biological processes .
Mode of action
This refers to how the compound interacts with its target. It could inhibit the target’s activity, enhance it, or modify it in some other way .
Biochemical pathways
These are sequences of chemical reactions that occur in a cell. The compound could affect one or more steps in these pathways, leading to changes in the cell’s behavior .
Pharmacokinetics
This refers to how the body affects a drug. It includes absorption (how the drug gets into the body), distribution (how it spreads throughout the body), metabolism (how it is chemically altered), and excretion (how it is removed from the body) .
Result of action
This is the outcome of the compound’s interaction with its target and its impact on biochemical pathways. It could involve changes in the cell’s function, the death of the cell, or other outcomes .
properties
IUPAC Name |
azidomethylcyclobutane | |
---|---|---|
Source | PubChem | |
URL | https://pubchem.ncbi.nlm.nih.gov | |
Description | Data deposited in or computed by PubChem | |
InChI |
InChI=1S/C5H9N3/c6-8-7-4-5-2-1-3-5/h5H,1-4H2 | |
Source | PubChem | |
URL | https://pubchem.ncbi.nlm.nih.gov | |
Description | Data deposited in or computed by PubChem | |
InChI Key |
JSTSIOABNCURKW-UHFFFAOYSA-N | |
Source | PubChem | |
URL | https://pubchem.ncbi.nlm.nih.gov | |
Description | Data deposited in or computed by PubChem | |
Canonical SMILES |
C1CC(C1)CN=[N+]=[N-] | |
Source | PubChem | |
URL | https://pubchem.ncbi.nlm.nih.gov | |
Description | Data deposited in or computed by PubChem | |
Molecular Formula |
C5H9N3 | |
Source | PubChem | |
URL | https://pubchem.ncbi.nlm.nih.gov | |
Description | Data deposited in or computed by PubChem | |
Molecular Weight |
111.15 g/mol | |
Source | PubChem | |
URL | https://pubchem.ncbi.nlm.nih.gov | |
Description | Data deposited in or computed by PubChem | |
Retrosynthesis Analysis
AI-Powered Synthesis Planning: Our tool employs the Template_relevance Pistachio, Template_relevance Bkms_metabolic, Template_relevance Pistachio_ringbreaker, Template_relevance Reaxys, Template_relevance Reaxys_biocatalysis model, leveraging a vast database of chemical reactions to predict feasible synthetic routes.
One-Step Synthesis Focus: Specifically designed for one-step synthesis, it provides concise and direct routes for your target compounds, streamlining the synthesis process.
Accurate Predictions: Utilizing the extensive PISTACHIO, BKMS_METABOLIC, PISTACHIO_RINGBREAKER, REAXYS, REAXYS_BIOCATALYSIS database, our tool offers high-accuracy predictions, reflecting the latest in chemical research and data.
Strategy Settings
Precursor scoring | Relevance Heuristic |
---|---|
Min. plausibility | 0.01 |
Model | Template_relevance |
Template Set | Pistachio/Bkms_metabolic/Pistachio_ringbreaker/Reaxys/Reaxys_biocatalysis |
Top-N result to add to graph | 6 |
Feasible Synthetic Routes
Disclaimer and Information on In-Vitro Research Products
Please be aware that all articles and product information presented on BenchChem are intended solely for informational purposes. The products available for purchase on BenchChem are specifically designed for in-vitro studies, which are conducted outside of living organisms. In-vitro studies, derived from the Latin term "in glass," involve experiments performed in controlled laboratory settings using cells or tissues. It is important to note that these products are not categorized as medicines or drugs, and they have not received approval from the FDA for the prevention, treatment, or cure of any medical condition, ailment, or disease. We must emphasize that any form of bodily introduction of these products into humans or animals is strictly prohibited by law. It is essential to adhere to these guidelines to ensure compliance with legal and ethical standards in research and experimentation.