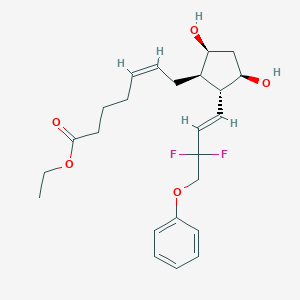
Tafluprost ethyl ester
Overview
Description
Tafluprost ethyl ester is a derivative of tafluprost, a prostaglandin F2α analog. Tafluprost is primarily used as an ocular hypotensive agent in the treatment of glaucoma and ocular hypertension. The ethyl ester form is a prodrug that is hydrolyzed to its active form, tafluprost acid, upon administration. This compound is known for its high efficacy in reducing intraocular pressure by increasing the outflow of aqueous humor from the eyes .
Mechanism of Action
Mode of Action
Tafluprost ethyl ester is an ester prodrug, which means it is converted into its active form, tafluprost acid, in the body . This conversion is facilitated by corneal esterases . Tafluprost acid is a prostanoid selective FP receptor agonist . It binds to the FP receptor with an affinity that is approximately 12 times higher than that of the carboxylic acid of latanoprost . This binding triggers an increase in the outflow of aqueous humor, which is believed to reduce intraocular pressure (IOP) .
Biochemical Pathways
The primary biochemical pathway involved in the action of this compound is the hydrolysis of the ester prodrug to form tafluprost acid, its biologically active metabolite . This process is facilitated by corneal esterases . Once activated, tafluprost acid is further metabolized via fatty acid β-oxidation and phase II conjugation into 1,2,3,4-tetranor acid .
Pharmacokinetics
As a lipophilic ester, this compound easily penetrates the cornea and is then activated to the carboxylic acid, tafluprost acid . The onset of action is 2 to 4 hours after application, the maximal effect is reached after 12 hours, and ocular pressure remains lowered for at least 24 hours . Tafluprost acid is inactivated by beta oxidation to 1,2-dinortafluprost acid, 1,2,3,4-tetranortafluprost acid, and its lactone, which are subsequently glucuronidated or hydroxylated .
Result of Action
The primary result of this compound’s action is a reduction in intraocular pressure (IOP). This is achieved by increasing the outflow of aqueous humor from the eyes . This makes it an effective treatment for conditions like ocular hypertension and open-angle glaucoma .
Action Environment
The action of this compound is influenced by the environment within the eye. As a lipophilic ester, it can easily penetrate the cornea, which is crucial for its activation and subsequent action
Biochemical Analysis
Biochemical Properties
Tafluprost ethyl ester acts as an agonist for the prostaglandin FP receptor. Upon hydrolysis by corneal esterases, it converts to tafluprost acid, which has a high affinity for the FP receptor. This interaction leads to the activation of signaling pathways that increase the outflow of aqueous humor from the eye, thereby reducing intraocular pressure . The compound interacts with enzymes such as esterases and proteins involved in the prostaglandin signaling pathway .
Cellular Effects
This compound influences various cellular processes primarily in ocular tissues. It affects cell signaling pathways by binding to the FP receptor, leading to changes in gene expression and cellular metabolism. The activation of the FP receptor by tafluprost acid results in the remodeling of the extracellular matrix, which facilitates increased aqueous humor outflow . Additionally, this compound has been shown to affect the expression of matrix metalloproteinases and tissue inhibitors of metalloproteinases in ocular cells .
Molecular Mechanism
At the molecular level, this compound is hydrolyzed to tafluprost acid, which then binds selectively to the prostaglandin FP receptor. This binding triggers a cascade of intracellular events, including the activation of G-proteins and subsequent signaling pathways that lead to increased uveoscleral outflow . The compound’s high affinity for the FP receptor ensures its potent ocular hypotensive effects .
Temporal Effects in Laboratory Settings
In laboratory settings, this compound demonstrates a stable and sustained effect on intraocular pressure reduction. The onset of action occurs within 2 to 4 hours after application, with the maximal effect observed at around 12 hours. The ocular pressure remains lowered for at least 24 hours . Studies have shown that this compound maintains its stability and efficacy over time, with minimal degradation observed in in vitro and in vivo studies .
Dosage Effects in Animal Models
In animal models, the effects of this compound vary with different dosages. Lower doses effectively reduce intraocular pressure without significant adverse effects, while higher doses may lead to toxic effects such as conjunctival hyperemia and respiratory issues . The compound has been shown to have a dose-dependent effect on ocular blood flow, with higher doses resulting in increased blood flow to the optic nerve head and retina .
Metabolic Pathways
This compound is metabolized through hydrolysis by corneal esterases to form tafluprost acid. The active metabolite is further metabolized via fatty acid β-oxidation and phase II conjugation into 1,2,3,4-tetranor acid . These metabolic pathways ensure the compound’s rapid activation and subsequent clearance from the body .
Transport and Distribution
This compound, being a lipophilic ester, easily penetrates the cornea and is distributed within ocular tissues. The compound is transported to the site of action, where it is hydrolyzed to tafluprost acid. The active metabolite is then distributed within the eye, exerting its effects on intraocular pressure . The transport and distribution of this compound are facilitated by its high lipid solubility, which enhances its uptake into tissues .
Subcellular Localization
This compound and its active metabolite, tafluprost acid, are localized primarily in the ocular tissues, particularly in the ciliary body and trabecular meshwork. The compound’s subcellular localization is directed by its lipophilic nature and the presence of specific targeting signals that guide it to the prostaglandin FP receptors . This localization is crucial for its therapeutic effects on intraocular pressure reduction .
Preparation Methods
Synthetic Routes and Reaction Conditions: The synthesis of tafluprost ethyl ester involves several key steps:
Oxidation: Starting from a suitable precursor, the compound undergoes oxidation to introduce necessary functional groups.
Condensation: The intermediate is then subjected to condensation reactions to form the core structure.
Fluorination: Introduction of fluorine atoms is achieved through specific fluorination reactions.
Deprotection and Reduction: Protective groups are removed, and the compound is reduced to achieve the desired stereochemistry.
Esterification: The final step involves esterification to form the ethyl ester derivative
Industrial Production Methods: Industrial production of this compound employs large-scale purification techniques such as reverse-phase and normal-phase column chromatography. The process also includes molecular group evacuation and the use of dispersing agents to enhance yield and purity .
Types of Reactions:
Oxidation: this compound can undergo oxidation to form various oxidized derivatives.
Reduction: Reduction reactions can convert the compound into different reduced forms.
Substitution: Substitution reactions can introduce different functional groups into the molecule.
Common Reagents and Conditions:
Oxidation: Reagents like hydrogen peroxide or potassium permanganate under acidic or basic conditions.
Reduction: Reagents such as lithium aluminum hydride or sodium borohydride.
Substitution: Reagents like halogens or alkylating agents under appropriate conditions
Major Products:
Oxidized Derivatives: Various oxidized forms depending on the specific oxidation conditions.
Reduced Forms: Different reduced forms based on the reducing agent used.
Substituted Compounds: Various substituted derivatives depending on the substituent introduced
Scientific Research Applications
Tafluprost ethyl ester has a wide range of applications in scientific research:
Chemistry: Used as a model compound for studying prostaglandin analogs and their derivatives.
Biology: Investigated for its effects on cellular processes and signaling pathways.
Medicine: Primarily used in the treatment of glaucoma and ocular hypertension. It is also studied for its potential in treating other eye-related conditions.
Industry: Employed in the development of new ophthalmic formulations and drug delivery systems
Comparison with Similar Compounds
Latanoprost: Another prostaglandin F2α analog used in the treatment of glaucoma.
Travoprost: Similar to latanoprost, used for reducing intraocular pressure.
Bimatoprost: An amide prodrug of a prostaglandin analog, also used for glaucoma treatment
Uniqueness: Tafluprost ethyl ester is unique due to its high affinity for the prostaglandin F receptor and its ability to be rapidly hydrolyzed to its active form. This results in a more potent and longer-lasting reduction in intraocular pressure compared to other similar compounds .
Properties
IUPAC Name |
ethyl (Z)-7-[(1R,2R,3R,5S)-2-[(E)-3,3-difluoro-4-phenoxybut-1-enyl]-3,5-dihydroxycyclopentyl]hept-5-enoate | |
---|---|---|
Source | PubChem | |
URL | https://pubchem.ncbi.nlm.nih.gov | |
Description | Data deposited in or computed by PubChem | |
InChI |
InChI=1S/C24H32F2O5/c1-2-30-23(29)13-9-4-3-8-12-19-20(22(28)16-21(19)27)14-15-24(25,26)17-31-18-10-6-5-7-11-18/h3,5-8,10-11,14-15,19-22,27-28H,2,4,9,12-13,16-17H2,1H3/b8-3-,15-14+/t19-,20-,21+,22-/m1/s1 | |
Source | PubChem | |
URL | https://pubchem.ncbi.nlm.nih.gov | |
Description | Data deposited in or computed by PubChem | |
InChI Key |
SPWJIEVPQKMQPO-MSHHKXPZSA-N | |
Source | PubChem | |
URL | https://pubchem.ncbi.nlm.nih.gov | |
Description | Data deposited in or computed by PubChem | |
Canonical SMILES |
CCOC(=O)CCCC=CCC1C(CC(C1C=CC(COC2=CC=CC=C2)(F)F)O)O | |
Source | PubChem | |
URL | https://pubchem.ncbi.nlm.nih.gov | |
Description | Data deposited in or computed by PubChem | |
Isomeric SMILES |
CCOC(=O)CCC/C=C\C[C@H]1[C@H](C[C@H]([C@@H]1/C=C/C(COC2=CC=CC=C2)(F)F)O)O | |
Source | PubChem | |
URL | https://pubchem.ncbi.nlm.nih.gov | |
Description | Data deposited in or computed by PubChem | |
Molecular Formula |
C24H32F2O5 | |
Source | PubChem | |
URL | https://pubchem.ncbi.nlm.nih.gov | |
Description | Data deposited in or computed by PubChem | |
Molecular Weight |
438.5 g/mol | |
Source | PubChem | |
URL | https://pubchem.ncbi.nlm.nih.gov | |
Description | Data deposited in or computed by PubChem | |
CAS No. |
209860-89-9 | |
Record name | Nortafluprost | |
Source | FDA Global Substance Registration System (GSRS) | |
URL | https://gsrs.ncats.nih.gov/ginas/app/beta/substances/WE6T2PLV6M | |
Description | The FDA Global Substance Registration System (GSRS) enables the efficient and accurate exchange of information on what substances are in regulated products. Instead of relying on names, which vary across regulatory domains, countries, and regions, the GSRS knowledge base makes it possible for substances to be defined by standardized, scientific descriptions. | |
Explanation | Unless otherwise noted, the contents of the FDA website (www.fda.gov), both text and graphics, are not copyrighted. They are in the public domain and may be republished, reprinted and otherwise used freely by anyone without the need to obtain permission from FDA. Credit to the U.S. Food and Drug Administration as the source is appreciated but not required. | |
Retrosynthesis Analysis
AI-Powered Synthesis Planning: Our tool employs the Template_relevance Pistachio, Template_relevance Bkms_metabolic, Template_relevance Pistachio_ringbreaker, Template_relevance Reaxys, Template_relevance Reaxys_biocatalysis model, leveraging a vast database of chemical reactions to predict feasible synthetic routes.
One-Step Synthesis Focus: Specifically designed for one-step synthesis, it provides concise and direct routes for your target compounds, streamlining the synthesis process.
Accurate Predictions: Utilizing the extensive PISTACHIO, BKMS_METABOLIC, PISTACHIO_RINGBREAKER, REAXYS, REAXYS_BIOCATALYSIS database, our tool offers high-accuracy predictions, reflecting the latest in chemical research and data.
Strategy Settings
Precursor scoring | Relevance Heuristic |
---|---|
Min. plausibility | 0.01 |
Model | Template_relevance |
Template Set | Pistachio/Bkms_metabolic/Pistachio_ringbreaker/Reaxys/Reaxys_biocatalysis |
Top-N result to add to graph | 6 |
Feasible Synthetic Routes
Disclaimer and Information on In-Vitro Research Products
Please be aware that all articles and product information presented on BenchChem are intended solely for informational purposes. The products available for purchase on BenchChem are specifically designed for in-vitro studies, which are conducted outside of living organisms. In-vitro studies, derived from the Latin term "in glass," involve experiments performed in controlled laboratory settings using cells or tissues. It is important to note that these products are not categorized as medicines or drugs, and they have not received approval from the FDA for the prevention, treatment, or cure of any medical condition, ailment, or disease. We must emphasize that any form of bodily introduction of these products into humans or animals is strictly prohibited by law. It is essential to adhere to these guidelines to ensure compliance with legal and ethical standards in research and experimentation.