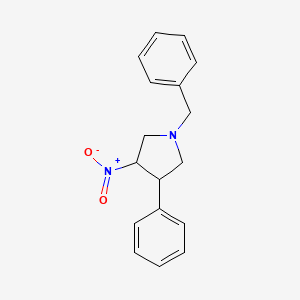
1-Benzyl-3-nitro-4-phenylpyrrolidine
Overview
Description
1-Benzyl-3-nitro-4-phenylpyrrolidine (CAS: 1823551-60-5) is a nitro-substituted pyrrolidine derivative with the molecular formula C₁₇H₁₈N₂O₂ and a molecular weight of 282.34 g/mol . Its structure features a benzyl group at position 1, a nitro group at position 3, and a phenyl ring at position 4 of the pyrrolidine scaffold.
Preparation Methods
Preparation via Dipolar Cycloaddition Using Flow Microreactor Technology
Overview
A prominent method for synthesizing 3-nitropyrrolidines, including 1-Benzyl-3-nitro-4-phenylpyrrolidine, is through a [3+2] dipolar cycloaddition reaction between azomethine ylids and nitroalkenes. This approach has been enhanced by employing flow microreactor technology, which offers improved heat and mass transfer, safer handling of hazardous reagents, and scalability from milligram to gram quantities.
Reaction Setup and Conditions
- Starting Materials:
- N-(methoxymethyl)-N-(trimethylsilyl)benzylamine as the dipole precursor.
- Nitroalkenes as the dipolarophile.
- Solvent: Acetonitrile (MeCN), typically 0.5 M concentration.
- Catalyst: Trifluoroacetic acid (TFA) used to activate the dipole precursor.
- Flow Reactor: Vapourtec R2+/R4 system with a heated coil reactor (10 mL volume).
- Temperature: 60–120 °C.
- Residence Time: 30–90 minutes.
- Purification: The reaction output is passed through a column containing immobilized benzylamine scavenger (QP-BZA) to remove excess nitroalkene, followed by silica gel to remove colored impurities.
Reaction Mechanism and Outcomes
- The azomethine ylid is generated in situ upon acid activation.
- The dipole reacts regioselectively with the nitroalkene to form the 3-nitropyrrolidine ring.
- The reaction proceeds with good yields (>70%) and purity after solvent removal.
- Diastereomeric mixtures are typically formed, with three main diastereoisomers observed under thermal conditions.
- Epimerization of stereocenters adjacent to the nitro group can occur during the reaction, leading to additional diastereomers.
Advantages
- Rapid synthesis with controlled reaction parameters.
- Improved safety and reproducibility via flow chemistry.
- Simplified purification using in-line scavengers.
- Suitable for scale-up and diverse substitution patterns on the pyrrolidine ring.
Parameter | Specification |
---|---|
Solvent | Acetonitrile (MeCN) |
Dipole Precursor | N-(methoxymethyl)-N-(trimethylsilyl)benzylamine |
Dipolarophile | Nitroalkene (varied substitution) |
Catalyst | Trifluoroacetic acid (TFA) |
Temperature Range | 60–120 °C |
Residence Time | 30–90 minutes |
Purification | Benzylamine scavenger column + silica gel plug |
Yield | >70% |
Source: Stanford research on flow microreactor synthesis of 3-nitropyrrolidines
Synthesis of 4-Phenylpyrrolidine Intermediates Relevant to this compound
While direct preparation of this compound is achieved via cycloaddition, related pyrrolidine derivatives such as 4-phenylpyrrolidone are synthesized through multi-step processes involving alkylation, catalytic hydrogenation, and cyclization. These intermediates provide insight into the preparation of phenyl-substituted pyrrolidines.
Key Steps in 4-Phenylpyrrolidone Synthesis
- Alkylation: Reaction of benzyl cyanide with ethyl bromoacetate under basic conditions (NaH, KOH, or NaOH) in solvents like tetrahydrofuran (THF) to form 3-cyano-ethyl phenylpropionate.
- Catalytic Hydrogenation: Raney-Nickel catalyst is used to hydrogenate the nitrile to the corresponding amine (4-amino-3-phenylbutyrate).
- Cyclization: The amino ester undergoes self-condensation under reflux to form 4-phenylpyrrolidone.
Reaction Conditions and Yields
Step | Reagents/Conditions | Yield (%) | Notes |
---|---|---|---|
Alkylation | Benzyl cyanide + ethyl bromoacetate, NaH, THF, RT, 8 h | 58.3–82 | Molar ratios and base affect yield |
Catalytic Hydrogenation | Raney-Ni, ethanol, H2 (1 atm), 4 h | 71 | Efficient reduction step |
Cyclization | Reflux, self-condensation | Not specified | Produces 4-phenylpyrrolidone |
Optimization Highlights
- Molar ratios of reagents are critical for maximizing yield and purity.
- Sodium hydride (NaH) generally provides better yields than KOH or NaOH.
- The Raney-Ni catalyst loading is optimized to minimize consumption while maintaining yield.
- The process is amenable to one-pot multistep synthesis, reducing solvent use and simplifying purification.
Source: Chinese patent CN103408480A on 4-phenylpyrrolidone synthesis
Comparative Analysis and Research Findings
Preparation Method | Advantages | Limitations |
---|---|---|
Flow Microreactor Dipolar Cycloaddition | Fast, scalable, safe, high purity, good yields | Diastereomeric mixtures, epimerization issues |
Multi-step Alkylation-Hydrogenation-Cyclization | Uses readily available materials, mild conditions, industrially scalable | Longer process, multiple purification steps |
Research Insights
- Flow chemistry enables rapid access to nitropyrrolidine derivatives with fine control over reaction parameters, crucial for pharmaceutical applications.
- Epimerization during thermal cycloaddition must be managed to control stereochemistry.
- The alkylation-hydrogenation-cyclization route provides a robust alternative for producing phenyl-substituted pyrrolidines, serving as intermediates for further functionalization.
- Both methods emphasize the importance of reaction condition optimization for yield and purity.
Chemical Reactions Analysis
1-Benzyl-3-nitro-4-phenylpyrrolidine undergoes various chemical reactions, including:
Oxidation: The nitro group can be reduced to an amino group using reducing agents such as hydrogen gas in the presence of a palladium catalyst.
Reduction: The compound can be oxidized to form corresponding N-oxides using oxidizing agents like m-chloroperbenzoic acid.
Substitution: The benzyl group can be substituted with other alkyl or aryl groups through nucleophilic substitution reactions.
Scientific Research Applications
Pharmaceutical Development
1-Benzyl-3-nitro-4-phenylpyrrolidine serves as an important intermediate in the synthesis of various pharmaceuticals, especially those targeting neurological disorders. Its structural properties allow for the development of drugs with enhanced efficacy against conditions such as depression and anxiety. Research indicates that this compound can influence neurotransmitter systems, offering potential therapeutic benefits in treating mood disorders .
Case Study: Anti-Alzheimer's Activity
A recent study explored the anti-Alzheimer's activity of this compound alongside other heterocyclic compounds. The compound demonstrated favorable binding affinities in silico against acetylcholinesterase, indicating potential as an anti-cholinesterase agent . This suggests its utility in combating neurodegenerative diseases by inhibiting enzyme activity that contributes to cognitive decline.
Neuroscience Research
Researchers utilize this compound to study its effects on neurotransmitter systems. This research is pivotal for understanding the underlying mechanisms of various psychological conditions and developing targeted therapies. The compound's interaction with specific receptors can lead to insights into treatment strategies for mental health disorders .
Organic Synthesis
In organic chemistry, this compound is employed as a versatile building block for synthesizing complex molecules. Its ability to undergo various chemical transformations makes it valuable for creating new compounds with desired properties. The synthesis typically involves nucleophilic substitution reactions using starting materials like benzyl bromide and nitrobenzene.
Material Science
The properties of this compound also lend themselves to applications in material science. It can be utilized in developing advanced materials, including polymers that exhibit specific mechanical or thermal characteristics beneficial for industrial applications .
Analytical Chemistry
In analytical chemistry, this compound is used as a standard in various methods to calibrate instruments and ensure accurate measurements. Its consistent chemical behavior allows researchers to establish reliable protocols for chemical analyses .
The biological activities associated with this compound include:
Activity Type | Description |
---|---|
Anticancer | Exhibits dose-dependent inhibition of cell proliferation in melanoma studies. |
Antileishmanial | Significant activity against Leishmania species in vitro. |
Neuroprotective | Potential to mitigate neuronal damage in neurodegenerative disease models. |
Mechanism of Action
The mechanism of action of 1-Benzyl-3-nitro-4-phenylpyrrolidine involves its interaction with specific molecular targets:
Comparison with Similar Compounds
Key Properties :
- Purity: Typically ≥95% (racemic mixture noted in some batches) .
- Storage: No specific storage guidelines are provided, indicating moderate stability under standard conditions .
- Availability : Sourced from China, the US, India, and Germany, though stock shortages are common .
Comparative Analysis with Structurally Similar Compounds
Structural Analogues in the Pyrrolidine/Piperidine Family
Table 1: Structural and Functional Group Comparisons
Key Observations :
- Functional Group Impact : The nitro group in this compound enhances electrophilicity compared to hydroxyl or hydroxymethyl substituents in analogues like (3S,4S)-1-Benzyl-3,4-pyrrolidindiol . This difference influences reactivity in synthetic pathways, such as nucleophilic substitutions or catalytic reductions.
- Stereoisomerism: The racemic mixture (rac-(3S,4R)) noted in some batches contrasts with the trans-isomer (QA-8474, CAS: 756791-42-1) listed by Combi-Blocks . Stereochemical variations may affect biological activity or crystallization behavior.
Comparison with Nitro-Containing Heterocycles
Key Observations :
- Reactivity : The nitro group at position 3 in this compound enables participation in [1,3]-dipolar cycloadditions, unlike nitro groups in alkenes (e.g., Trans-beta-nitrostyrene) .
- Pharmaceutical Relevance : Unlike Trans-4-Nitrostilbene (used in photochemistry), the target compound is linked to kinase inhibitor development, as seen in analogues like N-[(3RS,4RS)-1-Benzyl-4-methyl-piperidin-3-yl]-5-nitro... (a p38 MAP kinase inhibitor) .
Biological Activity
1-Benzyl-3-nitro-4-phenylpyrrolidine is a compound that has garnered attention in medicinal chemistry due to its potential biological activities. This article reviews the compound's structural characteristics, biological effects, and therapeutic implications based on available literature and research findings.
Structural Characteristics
This compound features a pyrrolidine ring substituted with a benzyl group and a nitro group at the 3-position. Its molecular formula is with a molar mass of approximately 286.34 g/mol. The presence of the nitro group is significant as it can influence the compound's reactivity and biological interactions.
The biological activity of this compound has been linked to its ability to interact with various cellular pathways, particularly those involved in cancer progression. Research indicates that this compound may act as an inhibitor of the ERK (extracellular signal-regulated kinase) signaling pathway, which is often upregulated in numerous malignancies, including melanoma, thyroid cancer, and colorectal cancer .
Key Findings:
- ERK Inhibition : The compound has shown potential as an ERK2 inhibitor, which could be beneficial in treating cancers associated with aberrant ERK signaling .
- Cytotoxicity : Preliminary studies suggest that it exhibits cytotoxic effects against certain cancer cell lines, indicating its potential as an anticancer agent .
Biological Activity Data
The following table summarizes some of the biological activities associated with this compound and related compounds:
Case Studies
- Cancer Research : In studies focused on melanoma, this compound was evaluated for its ability to inhibit cell proliferation. Results indicated a dose-dependent response in reducing cell viability, suggesting its utility as a therapeutic agent against resistant cancer types .
- Antileishmanial Activity : In vitro studies demonstrated that derivatives similar to this compound exhibited significant activity against both promastigote and amastigote forms of Leishmania, highlighting its potential in treating leishmaniasis .
- Neuroprotective Studies : Research investigating neurodegenerative diseases indicated that this compound could mitigate neuronal damage, suggesting further exploration into its neuroprotective properties .
Q & A
Basic Research Questions
Q. What synthetic routes are recommended for preparing 1-Benzyl-3-nitro-4-phenylpyrrolidine with high purity?
- Methodological Answer : A common approach involves multi-step nitro-group introduction via nitration reactions under controlled conditions (e.g., HNO₃/H₂SO₄). Purification via column chromatography (silica gel, eluent: ethyl acetate/hexane) is critical, with purity validation using HPLC (C18 column, UV detection at 254 nm). Evidence from rac-(3S,4R)-1-benzyl-3-nitro-4-phenylpyrrolidine synthesis highlights 95% purity achieved through recrystallization in ethanol . Alternative routes using piperidine intermediates (e.g., 1-benzyl-4-phenylamino-4-piperidinecarboxamide) may require Boc-protection strategies to avoid side reactions .
Q. Which spectroscopic techniques are most effective for structural characterization?
- Methodological Answer :
- X-ray crystallography : Resolves stereochemistry and bond angles (e.g., mean C–C bond length: 0.002 Å, R factor: 0.039) .
- NMR : ¹H and ¹³C NMR in CDCl₃ or DMSO-d₆ to assign benzyl, phenyl, and nitro-group signals. For example, the nitro group’s deshielding effect shifts protons on adjacent carbons to δ 4.2–4.5 ppm .
- IR spectroscopy : Confirm nitro-group presence via asymmetric stretching at ~1520 cm⁻¹ and symmetric stretching at ~1350 cm⁻¹ .
Q. How should researchers handle safety considerations during synthesis?
- Methodological Answer : Follow SDS guidelines for nitration reactions (e.g., use fume hoods, wear nitrile gloves). For this compound, avoid open flames due to potential exothermic decomposition. Store at 2–8°C under inert gas (N₂/Ar) to prevent nitro-group reduction .
Q. What solvents and conditions optimize solubility for reactivity studies?
- Methodological Answer : Polar aprotic solvents (e.g., DMF, DMSO) enhance solubility for SN2 reactions. For nucleophilic substitutions, use anhydrous conditions with molecular sieves. Solubility in ethanol (mp 95°C) suggests utility in recrystallization .
Advanced Research Questions
Q. How can contradictions in reported reaction yields (e.g., 60% vs. 85%) be resolved?
- Methodological Answer :
- Design of Experiments (DoE) : Vary parameters (temperature, catalyst loading) systematically. For example, compare Pd/C vs. Raney Ni in hydrogenation steps .
- Side-reaction analysis : Use LC-MS to detect byproducts (e.g., de-nitro derivatives) from over-reduction.
- Reproducibility protocols : Standardize solvent drying (e.g., MgSO₄ vs. 3Å molecular sieves) and monitor reaction progress via TLC .
Q. What computational strategies predict regioselectivity in nitro-group functionalization?
- Methodological Answer :
- DFT calculations : Use Gaussian or ORCA to model transition states. For example, calculate activation energies for nitro-group reduction pathways.
- Docking studies : If targeting biological activity, simulate interactions with enzymes (e.g., cytochrome P450) to prioritize synthetic modifications .
Q. How can enantiomeric excess (ee) be improved in asymmetric synthesis?
- Methodological Answer :
- Chiral catalysts : Test Jacobsen’s Mn-salen complexes or BINOL-derived phosphoric acids for kinetic resolution.
- Chiral HPLC : Use Chiralpak IA/IB columns to quantify ee. For example, baseline separation of (3S,4R) and (3R,4S) enantiomers may require hexane/isopropanol gradients .
Q. What mechanistic insights explain unexpected thermal instability during storage?
- Methodological Answer :
- TGA/DSC analysis : Identify decomposition onset temperatures. For example, nitro compounds often degrade above 150°C.
- Accelerated aging studies : Store samples at 40°C/75% RH for 4 weeks and monitor purity via HPLC. Degradation products (e.g., benzaldehyde) suggest hydrolysis of the benzyl group .
Q. Notes for Methodological Rigor
Properties
IUPAC Name |
1-benzyl-3-nitro-4-phenylpyrrolidine | |
---|---|---|
Source | PubChem | |
URL | https://pubchem.ncbi.nlm.nih.gov | |
Description | Data deposited in or computed by PubChem | |
InChI |
InChI=1S/C17H18N2O2/c20-19(21)17-13-18(11-14-7-3-1-4-8-14)12-16(17)15-9-5-2-6-10-15/h1-10,16-17H,11-13H2 | |
Source | PubChem | |
URL | https://pubchem.ncbi.nlm.nih.gov | |
Description | Data deposited in or computed by PubChem | |
InChI Key |
BVUNFAAOQWGVQX-UHFFFAOYSA-N | |
Source | PubChem | |
URL | https://pubchem.ncbi.nlm.nih.gov | |
Description | Data deposited in or computed by PubChem | |
Canonical SMILES |
C1C(C(CN1CC2=CC=CC=C2)[N+](=O)[O-])C3=CC=CC=C3 | |
Source | PubChem | |
URL | https://pubchem.ncbi.nlm.nih.gov | |
Description | Data deposited in or computed by PubChem | |
Molecular Formula |
C17H18N2O2 | |
Source | PubChem | |
URL | https://pubchem.ncbi.nlm.nih.gov | |
Description | Data deposited in or computed by PubChem | |
Molecular Weight |
282.34 g/mol | |
Source | PubChem | |
URL | https://pubchem.ncbi.nlm.nih.gov | |
Description | Data deposited in or computed by PubChem | |
Synthesis routes and methods I
Procedure details
Synthesis routes and methods II
Procedure details
Retrosynthesis Analysis
AI-Powered Synthesis Planning: Our tool employs the Template_relevance Pistachio, Template_relevance Bkms_metabolic, Template_relevance Pistachio_ringbreaker, Template_relevance Reaxys, Template_relevance Reaxys_biocatalysis model, leveraging a vast database of chemical reactions to predict feasible synthetic routes.
One-Step Synthesis Focus: Specifically designed for one-step synthesis, it provides concise and direct routes for your target compounds, streamlining the synthesis process.
Accurate Predictions: Utilizing the extensive PISTACHIO, BKMS_METABOLIC, PISTACHIO_RINGBREAKER, REAXYS, REAXYS_BIOCATALYSIS database, our tool offers high-accuracy predictions, reflecting the latest in chemical research and data.
Strategy Settings
Precursor scoring | Relevance Heuristic |
---|---|
Min. plausibility | 0.01 |
Model | Template_relevance |
Template Set | Pistachio/Bkms_metabolic/Pistachio_ringbreaker/Reaxys/Reaxys_biocatalysis |
Top-N result to add to graph | 6 |
Feasible Synthetic Routes
Disclaimer and Information on In-Vitro Research Products
Please be aware that all articles and product information presented on BenchChem are intended solely for informational purposes. The products available for purchase on BenchChem are specifically designed for in-vitro studies, which are conducted outside of living organisms. In-vitro studies, derived from the Latin term "in glass," involve experiments performed in controlled laboratory settings using cells or tissues. It is important to note that these products are not categorized as medicines or drugs, and they have not received approval from the FDA for the prevention, treatment, or cure of any medical condition, ailment, or disease. We must emphasize that any form of bodily introduction of these products into humans or animals is strictly prohibited by law. It is essential to adhere to these guidelines to ensure compliance with legal and ethical standards in research and experimentation.