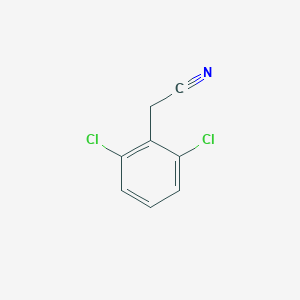
2,6-Dichlorobenzyl cyanide
Overview
Description
2,6-Dichlorobenzyl cyanide, also known as 2,6-Dichlorophenylacetonitrile, is a chemical compound with the linear formula Cl2C6H3CH2CN . It has a molecular weight of 186.04 .
Molecular Structure Analysis
The molecular structure of 2,6-Dichlorobenzyl cyanide is represented by the linear formula Cl2C6H3CH2CN . This indicates that the molecule consists of a benzyl group (C6H3CH2) with two chlorine atoms (Cl2) and a cyanide group (CN).Physical And Chemical Properties Analysis
2,6-Dichlorobenzyl cyanide is a solid at 20 degrees Celsius . It has a melting point of 73-75 °C .Scientific Research Applications
Soil Degradation Study
2,6-Dichlorophenylacetonitrile has been found to degrade rapidly in non-sterile soils . This property makes it a useful compound in environmental studies, particularly in understanding the degradation process of chemicals in soil environments. The degradation rate of this compound in soil can provide insights into the soil’s microbial activity and its ability to break down various substances.
Inhibition of Carbonyl Reductase Enzyme
The compound has been synthesized and characterized using a variety of physical, electrochemical, and spectroscopic methods . It has been found to be a powerful inhibitor of the Carbonyl Reductase enzyme . This enzyme is involved in the development of resistance to anticancer treatment, and the making of cardiotoxic derivatives of anthracyclines that are currently used in medicine . Therefore, 2,6-Dichlorophenylacetonitrile could potentially be used in cancer research and treatment strategies.
Safety And Hazards
2,6-Dichlorobenzyl cyanide is classified as Acute Tox. 4 for oral, dermal, and inhalation routes. It is also classified as Eye Irrit. 2 and Skin Irrit. 2, indicating that it causes skin irritation and serious eye irritation . Safety measures include avoiding dust formation, avoiding breathing vapors, mist or gas, ensuring adequate ventilation, and using personal protective equipment .
properties
IUPAC Name |
2-(2,6-dichlorophenyl)acetonitrile | |
---|---|---|
Source | PubChem | |
URL | https://pubchem.ncbi.nlm.nih.gov | |
Description | Data deposited in or computed by PubChem | |
InChI |
InChI=1S/C8H5Cl2N/c9-7-2-1-3-8(10)6(7)4-5-11/h1-3H,4H2 | |
Source | PubChem | |
URL | https://pubchem.ncbi.nlm.nih.gov | |
Description | Data deposited in or computed by PubChem | |
InChI Key |
AOEJUUCUKRUCEF-UHFFFAOYSA-N | |
Source | PubChem | |
URL | https://pubchem.ncbi.nlm.nih.gov | |
Description | Data deposited in or computed by PubChem | |
Canonical SMILES |
C1=CC(=C(C(=C1)Cl)CC#N)Cl | |
Source | PubChem | |
URL | https://pubchem.ncbi.nlm.nih.gov | |
Description | Data deposited in or computed by PubChem | |
Molecular Formula |
C8H5Cl2N | |
Source | PubChem | |
URL | https://pubchem.ncbi.nlm.nih.gov | |
Description | Data deposited in or computed by PubChem | |
DSSTOX Substance ID |
DTXSID00185922 | |
Record name | (2,6-Dichlorophenyl)acetonitrile | |
Source | EPA DSSTox | |
URL | https://comptox.epa.gov/dashboard/DTXSID00185922 | |
Description | DSSTox provides a high quality public chemistry resource for supporting improved predictive toxicology. | |
Molecular Weight |
186.03 g/mol | |
Source | PubChem | |
URL | https://pubchem.ncbi.nlm.nih.gov | |
Description | Data deposited in or computed by PubChem | |
Product Name |
2,6-Dichlorobenzyl cyanide | |
CAS RN |
3215-64-3 | |
Record name | 2,6-Dichlorobenzeneacetonitrile | |
Source | CAS Common Chemistry | |
URL | https://commonchemistry.cas.org/detail?cas_rn=3215-64-3 | |
Description | CAS Common Chemistry is an open community resource for accessing chemical information. Nearly 500,000 chemical substances from CAS REGISTRY cover areas of community interest, including common and frequently regulated chemicals, and those relevant to high school and undergraduate chemistry classes. This chemical information, curated by our expert scientists, is provided in alignment with our mission as a division of the American Chemical Society. | |
Explanation | The data from CAS Common Chemistry is provided under a CC-BY-NC 4.0 license, unless otherwise stated. | |
Record name | (2,6-Dichlorophenyl)acetonitrile | |
Source | ChemIDplus | |
URL | https://pubchem.ncbi.nlm.nih.gov/substance/?source=chemidplus&sourceid=0003215643 | |
Description | ChemIDplus is a free, web search system that provides access to the structure and nomenclature authority files used for the identification of chemical substances cited in National Library of Medicine (NLM) databases, including the TOXNET system. | |
Record name | 2,6-Dichlorobenzyl cyanide | |
Source | DTP/NCI | |
URL | https://dtp.cancer.gov/dtpstandard/servlet/dwindex?searchtype=NSC&outputformat=html&searchlist=72901 | |
Description | The NCI Development Therapeutics Program (DTP) provides services and resources to the academic and private-sector research communities worldwide to facilitate the discovery and development of new cancer therapeutic agents. | |
Explanation | Unless otherwise indicated, all text within NCI products is free of copyright and may be reused without our permission. Credit the National Cancer Institute as the source. | |
Record name | (2,6-Dichlorophenyl)acetonitrile | |
Source | EPA DSSTox | |
URL | https://comptox.epa.gov/dashboard/DTXSID00185922 | |
Description | DSSTox provides a high quality public chemistry resource for supporting improved predictive toxicology. | |
Record name | (2,6-dichlorophenyl)acetonitrile | |
Source | European Chemicals Agency (ECHA) | |
URL | https://echa.europa.eu/substance-information/-/substanceinfo/100.019.755 | |
Description | The European Chemicals Agency (ECHA) is an agency of the European Union which is the driving force among regulatory authorities in implementing the EU's groundbreaking chemicals legislation for the benefit of human health and the environment as well as for innovation and competitiveness. | |
Explanation | Use of the information, documents and data from the ECHA website is subject to the terms and conditions of this Legal Notice, and subject to other binding limitations provided for under applicable law, the information, documents and data made available on the ECHA website may be reproduced, distributed and/or used, totally or in part, for non-commercial purposes provided that ECHA is acknowledged as the source: "Source: European Chemicals Agency, http://echa.europa.eu/". Such acknowledgement must be included in each copy of the material. ECHA permits and encourages organisations and individuals to create links to the ECHA website under the following cumulative conditions: Links can only be made to webpages that provide a link to the Legal Notice page. | |
Record name | 2,6-Dichlorobenzeneacetonitrile | |
Source | FDA Global Substance Registration System (GSRS) | |
URL | https://gsrs.ncats.nih.gov/ginas/app/beta/substances/YDY3AJ66GX | |
Description | The FDA Global Substance Registration System (GSRS) enables the efficient and accurate exchange of information on what substances are in regulated products. Instead of relying on names, which vary across regulatory domains, countries, and regions, the GSRS knowledge base makes it possible for substances to be defined by standardized, scientific descriptions. | |
Explanation | Unless otherwise noted, the contents of the FDA website (www.fda.gov), both text and graphics, are not copyrighted. They are in the public domain and may be republished, reprinted and otherwise used freely by anyone without the need to obtain permission from FDA. Credit to the U.S. Food and Drug Administration as the source is appreciated but not required. | |
Synthesis routes and methods
Procedure details
Retrosynthesis Analysis
AI-Powered Synthesis Planning: Our tool employs the Template_relevance Pistachio, Template_relevance Bkms_metabolic, Template_relevance Pistachio_ringbreaker, Template_relevance Reaxys, Template_relevance Reaxys_biocatalysis model, leveraging a vast database of chemical reactions to predict feasible synthetic routes.
One-Step Synthesis Focus: Specifically designed for one-step synthesis, it provides concise and direct routes for your target compounds, streamlining the synthesis process.
Accurate Predictions: Utilizing the extensive PISTACHIO, BKMS_METABOLIC, PISTACHIO_RINGBREAKER, REAXYS, REAXYS_BIOCATALYSIS database, our tool offers high-accuracy predictions, reflecting the latest in chemical research and data.
Strategy Settings
Precursor scoring | Relevance Heuristic |
---|---|
Min. plausibility | 0.01 |
Model | Template_relevance |
Template Set | Pistachio/Bkms_metabolic/Pistachio_ringbreaker/Reaxys/Reaxys_biocatalysis |
Top-N result to add to graph | 6 |
Feasible Synthetic Routes
Q & A
Q1: How does the substitution at the 7-position of 3-(2,6-dichlorophenyl)-1,6-naphthyridin-2(1H)-one, derived from 2,6-Dichlorophenylacetonitrile, impact its inhibitory activity against c-Src kinase?
A1: Research indicates that introducing basic aliphatic side chains at the 7-position of 3-(2,6-dichlorophenyl)-1,6-naphthyridin-2(1H)-one significantly enhances its inhibitory activity against c-Src kinase. Compounds with 7-NH(CH2)nNRR, 7-NHPhO(CH2)nNRR, or 7-NHPhN(CH2)4NMe substituents displayed the most potent inhibition, with IC50 values in the 10-80 nM range. This potency is attributed to the basic side chains interacting favorably with the enzyme's active site. [] Interestingly, these compounds also exhibited selectivity for c-Src over PDGFR, highlighting the importance of the 7-position substitution for achieving both potency and selectivity. []
Disclaimer and Information on In-Vitro Research Products
Please be aware that all articles and product information presented on BenchChem are intended solely for informational purposes. The products available for purchase on BenchChem are specifically designed for in-vitro studies, which are conducted outside of living organisms. In-vitro studies, derived from the Latin term "in glass," involve experiments performed in controlled laboratory settings using cells or tissues. It is important to note that these products are not categorized as medicines or drugs, and they have not received approval from the FDA for the prevention, treatment, or cure of any medical condition, ailment, or disease. We must emphasize that any form of bodily introduction of these products into humans or animals is strictly prohibited by law. It is essential to adhere to these guidelines to ensure compliance with legal and ethical standards in research and experimentation.