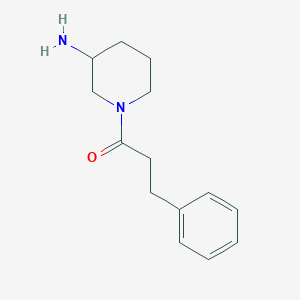
1-(3-Aminopiperidin-1-yl)-3-phenylpropan-1-one
Overview
Description
1-(3-Aminopiperidin-1-yl)-3-phenylpropan-1-one is a chemical compound that belongs to the class of piperidine derivatives. Piperidine is a six-membered heterocycle containing one nitrogen atom and five carbon atoms in the sp3-hybridized state
Mechanism of Action
Target of Action
The primary target of 1-(3-Aminopiperidin-1-yl)-3-phenylpropan-1-one is Dipeptidyl Peptidase-4 (DPP-4) . DPP-4 is an enzyme responsible for the degradation of incretin hormones, which are involved in the regulation of glucose metabolism .
Mode of Action
This compound interacts with its target, DPP-4, by inhibiting its activity . This inhibition results in an increased level of incretin hormones, which stimulate insulin secretion and inhibit glucagon release, thereby regulating blood glucose levels .
Biochemical Pathways
The inhibition of DPP-4 affects the incretin hormone pathway. Incretin hormones, such as Glucagon-Like Peptide-1 (GLP-1), are released in response to food intake and stimulate insulin secretion from pancreatic beta cells. By inhibiting DPP-4, the degradation of incretin hormones is reduced, leading to increased insulin secretion and decreased glucagon release. This results in lower blood glucose levels .
Pharmacokinetics
Similar compounds, such as dpp-4 inhibitors, are known to be readily absorbed orally, with absorption occurring mainly in the small intestine . The metabolism of these compounds is variable, with reported terminal half-lives ranging from approximately 3 to more than 200 hours .
Result of Action
The molecular and cellular effects of this compound’s action include increased levels of incretin hormones, increased insulin secretion, decreased glucagon release, and ultimately, the regulation of blood glucose levels .
Biochemical Analysis
Biochemical Properties
1-(3-Aminopiperidin-1-yl)-3-phenylpropan-1-one plays a significant role in biochemical reactions by interacting with various enzymes, proteins, and other biomolecules. It has been observed to interact with dipeptidyl peptidase-4 (DPP-4), an enzyme involved in glucose metabolism. The interaction between this compound and DPP-4 results in the inhibition of the enzyme’s activity, which can influence glucose homeostasis
Cellular Effects
This compound has been shown to affect various types of cells and cellular processes. In particular, it influences cell signaling pathways, gene expression, and cellular metabolism. For example, the inhibition of DPP-4 by this compound can lead to increased levels of glucagon-like peptide-1 (GLP-1), which in turn enhances insulin secretion and improves glucose tolerance
Molecular Mechanism
The molecular mechanism of action of this compound involves its binding interactions with biomolecules, enzyme inhibition, and changes in gene expression. By binding to the active site of DPP-4, this compound inhibits the enzyme’s activity, preventing the degradation of GLP-1 and other incretin hormones . This inhibition leads to increased levels of active GLP-1, which promotes insulin secretion and improves glucose homeostasis. Additionally, the compound may influence the expression of genes involved in metabolic pathways, further contributing to its biochemical effects.
Dosage Effects in Animal Models
The effects of this compound vary with different dosages in animal models. At lower doses, the compound effectively inhibits DPP-4 activity and improves glucose tolerance without significant adverse effects . At higher doses, toxic effects such as liver and kidney damage have been observed, indicating the importance of careful dosage optimization in therapeutic applications . Threshold effects and dose-response relationships need to be further explored to determine the optimal therapeutic window for this compound.
Metabolic Pathways
This compound is involved in several metabolic pathways, including those related to glucose and lipid metabolism. The compound interacts with enzymes such as DPP-4 and may influence the activity of other metabolic enzymes and cofactors These interactions can affect metabolic flux and metabolite levels, contributing to the compound’s overall biochemical effects
Transport and Distribution
The transport and distribution of this compound within cells and tissues are influenced by various transporters and binding proteins. The compound is likely to be distributed widely in tissues, with a preference for certain organs such as the liver and kidneys
Subcellular Localization
The subcellular localization of this compound can affect its activity and function. The compound may be directed to specific compartments or organelles through targeting signals or post-translational modifications . Understanding the subcellular localization of this compound is crucial for elucidating its precise mechanisms of action and potential therapeutic applications.
Preparation Methods
Synthetic Routes and Reaction Conditions
One common method involves the cyclization of amino alcohols with diols catalyzed by a Cp*Ir complex . Another approach is the one-pot preparation of cyclic amines via efficient chlorination of amino alcohols using SOCl2 . These methods provide good yields and are efficient for the synthesis of piperidine derivatives.
Industrial Production Methods
Industrial production of piperidine derivatives often involves multicomponent reactions and hydrogenation processes. The development of fast and cost-effective methods for the synthesis of substituted piperidines is an important task in modern organic chemistry . Continuous flow reactions and transfer hydrogenation protocols are commonly employed in industrial settings to produce these compounds on a large scale .
Chemical Reactions Analysis
Types of Reactions
1-(3-Aminopiperidin-1-yl)-3-phenylpropan-1-one undergoes various chemical reactions, including:
Oxidation: This compound can be oxidized using reagents such as potassium permanganate or chromium trioxide.
Substitution: Nucleophilic substitution reactions can occur at the piperidine nitrogen or the phenyl ring.
Common Reagents and Conditions
Oxidation: Potassium permanganate (KMnO4), chromium trioxide (CrO3)
Reduction: Palladium on carbon (Pd/C), borane-ammonia (H3N-BH3)
Substitution: Alkyl halides, acyl chlorides
Major Products Formed
Oxidation: Formation of ketones or carboxylic acids
Reduction: Formation of amines or alcohols
Substitution: Formation of substituted piperidines or phenyl derivatives
Scientific Research Applications
1-(3-Aminopiperidin-1-yl)-3-phenylpropan-1-one has a wide range of scientific research applications:
Comparison with Similar Compounds
Similar Compounds
Linagliptin: 8-[(3R)-3-aminopiperidin-1-yl]-7-but-2-ynyl-3-methyl-1-[(4-methylquinazolin-2-yl)methyl]purine-2,6-dione
Uniqueness
1-(3-Aminopiperidin-1-yl)-3-phenylpropan-1-one is unique due to its specific structural features and potential biological activities. Its piperidine ring and phenylpropanone moiety provide a versatile scaffold for the development of novel therapeutic agents. Additionally, its ability to undergo various chemical reactions makes it a valuable compound in synthetic chemistry.
Properties
IUPAC Name |
1-(3-aminopiperidin-1-yl)-3-phenylpropan-1-one | |
---|---|---|
Source | PubChem | |
URL | https://pubchem.ncbi.nlm.nih.gov | |
Description | Data deposited in or computed by PubChem | |
InChI |
InChI=1S/C14H20N2O/c15-13-7-4-10-16(11-13)14(17)9-8-12-5-2-1-3-6-12/h1-3,5-6,13H,4,7-11,15H2 | |
Source | PubChem | |
URL | https://pubchem.ncbi.nlm.nih.gov | |
Description | Data deposited in or computed by PubChem | |
InChI Key |
BOYBTHQIDZITNZ-UHFFFAOYSA-N | |
Source | PubChem | |
URL | https://pubchem.ncbi.nlm.nih.gov | |
Description | Data deposited in or computed by PubChem | |
Canonical SMILES |
C1CC(CN(C1)C(=O)CCC2=CC=CC=C2)N | |
Source | PubChem | |
URL | https://pubchem.ncbi.nlm.nih.gov | |
Description | Data deposited in or computed by PubChem | |
Molecular Formula |
C14H20N2O | |
Source | PubChem | |
URL | https://pubchem.ncbi.nlm.nih.gov | |
Description | Data deposited in or computed by PubChem | |
Molecular Weight |
232.32 g/mol | |
Source | PubChem | |
URL | https://pubchem.ncbi.nlm.nih.gov | |
Description | Data deposited in or computed by PubChem | |
Retrosynthesis Analysis
AI-Powered Synthesis Planning: Our tool employs the Template_relevance Pistachio, Template_relevance Bkms_metabolic, Template_relevance Pistachio_ringbreaker, Template_relevance Reaxys, Template_relevance Reaxys_biocatalysis model, leveraging a vast database of chemical reactions to predict feasible synthetic routes.
One-Step Synthesis Focus: Specifically designed for one-step synthesis, it provides concise and direct routes for your target compounds, streamlining the synthesis process.
Accurate Predictions: Utilizing the extensive PISTACHIO, BKMS_METABOLIC, PISTACHIO_RINGBREAKER, REAXYS, REAXYS_BIOCATALYSIS database, our tool offers high-accuracy predictions, reflecting the latest in chemical research and data.
Strategy Settings
Precursor scoring | Relevance Heuristic |
---|---|
Min. plausibility | 0.01 |
Model | Template_relevance |
Template Set | Pistachio/Bkms_metabolic/Pistachio_ringbreaker/Reaxys/Reaxys_biocatalysis |
Top-N result to add to graph | 6 |
Feasible Synthetic Routes
Disclaimer and Information on In-Vitro Research Products
Please be aware that all articles and product information presented on BenchChem are intended solely for informational purposes. The products available for purchase on BenchChem are specifically designed for in-vitro studies, which are conducted outside of living organisms. In-vitro studies, derived from the Latin term "in glass," involve experiments performed in controlled laboratory settings using cells or tissues. It is important to note that these products are not categorized as medicines or drugs, and they have not received approval from the FDA for the prevention, treatment, or cure of any medical condition, ailment, or disease. We must emphasize that any form of bodily introduction of these products into humans or animals is strictly prohibited by law. It is essential to adhere to these guidelines to ensure compliance with legal and ethical standards in research and experimentation.