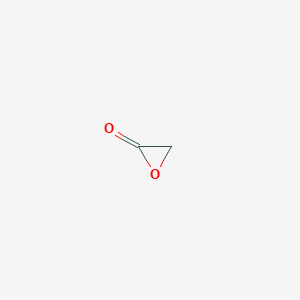
Acetolactone
Overview
Description
Acetolactone, also known as oxiran-2-one, is an organic compound with the molecular formula C₂H₂O₂. It is the smallest member of the lactone family and can also be described as the epoxide of ethenone. This compound was first described in 1997 as a transient species in mass spectrometry experiments .
Preparation Methods
Synthetic Routes and Reaction Conditions: Acetolactone can be synthesized from single linear molecules that contain both alcohol and carboxylic acid functionalities. For example, the preparation can be represented as: [ \text{HO-CH}_2\text{-COOH} \rightarrow \text{this compound} + \text{H}_2\text{O} ] This reaction involves the internal esterification of the molecule .
Industrial Production Methods: Although this compound itself has not been isolated in bulk, a related species, bis(trifluoromethyl)this compound, is known and has a half-life of 8 hours at 25°C. This compound is prepared by photolysis of bis(trifluoromethyl)malonyl peroxide .
Chemical Reactions Analysis
Types of Reactions: Acetolactone undergoes various chemical reactions, including:
Oxidation: this compound can be oxidized to form different products depending on the reagents and conditions used.
Reduction: It can be reduced to form simpler compounds.
Substitution: this compound can participate in substitution reactions where one of its atoms is replaced by another atom or group.
Common Reagents and Conditions:
Oxidation: Common oxidizing agents include potassium permanganate and chromium trioxide.
Reduction: Reducing agents such as lithium aluminum hydride can be used.
Substitution: Various nucleophiles can be used for substitution reactions.
Major Products: The major products formed from these reactions depend on the specific reagents and conditions used. For example, oxidation can lead to the formation of carboxylic acids, while reduction can yield alcohols.
Scientific Research Applications
Acetolactone has several applications in scientific research:
Chemistry: It is used as an intermediate in the synthesis of various organic compounds.
Biology: this compound can be used in studies involving enzyme reactions and metabolic pathways.
Industry: this compound is used in the production of polymers and other industrial chemicals.
Mechanism of Action
The mechanism by which acetolactone exerts its effects involves its reactivity as a lactone. It can undergo ring-opening reactions, which are crucial in various chemical processes. The molecular targets and pathways involved depend on the specific application and the conditions under which this compound is used .
Comparison with Similar Compounds
Butyrolactone: Gamma-butyrolactone is a more useful compound and is used as a reagent to produce other compounds and as a solvent.
Valerolactone: Delta-valerolactone is used in certain living, cationic polyester-formation reactions.
Uniqueness: Acetolactone is unique due to its small size and high reactivity. Its transient nature makes it a valuable compound for studying reaction mechanisms and intermediate species in mass spectrometry experiments .
Properties
IUPAC Name |
oxiran-2-one | |
---|---|---|
Details | Computed by LexiChem 2.6.6 (PubChem release 2019.06.18) | |
Source | PubChem | |
URL | https://pubchem.ncbi.nlm.nih.gov | |
Description | Data deposited in or computed by PubChem | |
InChI |
InChI=1S/C2H2O2/c3-2-1-4-2/h1H2 | |
Details | Computed by InChI 1.0.5 (PubChem release 2019.06.18) | |
Source | PubChem | |
URL | https://pubchem.ncbi.nlm.nih.gov | |
Description | Data deposited in or computed by PubChem | |
InChI Key |
HZSIFDFXFAXICF-UHFFFAOYSA-N | |
Details | Computed by InChI 1.0.5 (PubChem release 2019.06.18) | |
Source | PubChem | |
URL | https://pubchem.ncbi.nlm.nih.gov | |
Description | Data deposited in or computed by PubChem | |
Canonical SMILES |
C1C(=O)O1 | |
Details | Computed by OEChem 2.1.5 (PubChem release 2019.06.18) | |
Source | PubChem | |
URL | https://pubchem.ncbi.nlm.nih.gov | |
Description | Data deposited in or computed by PubChem | |
Molecular Formula |
C2H2O2 | |
Details | Computed by PubChem 2.1 (PubChem release 2019.06.18) | |
Source | PubChem | |
URL | https://pubchem.ncbi.nlm.nih.gov | |
Description | Data deposited in or computed by PubChem | |
DSSTOX Substance ID |
DTXSID30332189 | |
Record name | 2-Oxiranone | |
Source | EPA DSSTox | |
URL | https://comptox.epa.gov/dashboard/DTXSID30332189 | |
Description | DSSTox provides a high quality public chemistry resource for supporting improved predictive toxicology. | |
Molecular Weight |
58.04 g/mol | |
Details | Computed by PubChem 2.1 (PubChem release 2021.05.07) | |
Source | PubChem | |
URL | https://pubchem.ncbi.nlm.nih.gov | |
Description | Data deposited in or computed by PubChem | |
CAS No. |
42879-41-4 | |
Record name | 2-Oxiranone | |
Source | CAS Common Chemistry | |
URL | https://commonchemistry.cas.org/detail?cas_rn=42879-41-4 | |
Description | CAS Common Chemistry is an open community resource for accessing chemical information. Nearly 500,000 chemical substances from CAS REGISTRY cover areas of community interest, including common and frequently regulated chemicals, and those relevant to high school and undergraduate chemistry classes. This chemical information, curated by our expert scientists, is provided in alignment with our mission as a division of the American Chemical Society. | |
Explanation | The data from CAS Common Chemistry is provided under a CC-BY-NC 4.0 license, unless otherwise stated. | |
Record name | 2-Oxiranone | |
Source | EPA DSSTox | |
URL | https://comptox.epa.gov/dashboard/DTXSID30332189 | |
Description | DSSTox provides a high quality public chemistry resource for supporting improved predictive toxicology. | |
Disclaimer and Information on In-Vitro Research Products
Please be aware that all articles and product information presented on BenchChem are intended solely for informational purposes. The products available for purchase on BenchChem are specifically designed for in-vitro studies, which are conducted outside of living organisms. In-vitro studies, derived from the Latin term "in glass," involve experiments performed in controlled laboratory settings using cells or tissues. It is important to note that these products are not categorized as medicines or drugs, and they have not received approval from the FDA for the prevention, treatment, or cure of any medical condition, ailment, or disease. We must emphasize that any form of bodily introduction of these products into humans or animals is strictly prohibited by law. It is essential to adhere to these guidelines to ensure compliance with legal and ethical standards in research and experimentation.