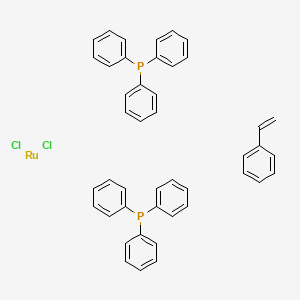
Benzylidene-bis(triphenylphosphine)ruthenium dichloride
- Click on QUICK INQUIRY to receive a quote from our team of experts.
- With the quality product at a COMPETITIVE price, you can focus more on your research.
Overview
Description
Benzylidene-bis(triphenylphosphine)ruthenium dichloride is a ruthenium-based coordination complex featuring two triphenylphosphine (PPh₃) ligands, a benzylidene carbene ligand, and two chloride counterions. This compound belongs to the family of Grubbs-type catalysts but differs from the first-generation Grubbs catalyst, which contains tricyclohexylphosphine (PCy₃) ligands instead of PPh₃. The substitution of PCy₃ with PPh₃ alters the steric and electronic properties of the complex, influencing its catalytic activity and selectivity in organic transformations such as olefin metathesis, hydrogenation, and atom-transfer radical polymerization (ATRP) .
Preparation Methods
Synthetic Routes and Reaction Conditions
Benzylidene-bis(triphenylphosphine)ruthenium dichloride is typically synthesized through the reaction of tricyclohexylphosphine with ruthenium trichloride hydrate in the presence of benzylidene chloride. The reaction is carried out under an inert atmosphere, usually nitrogen or argon, to prevent oxidation. The mixture is heated to reflux, and the product is purified by recrystallization .
Industrial Production Methods
In industrial settings, the production of this compound follows similar synthetic routes but on a larger scale. The process involves stringent control of reaction conditions to ensure high yield and purity. The use of automated reactors and continuous flow systems can enhance efficiency and scalability .
Chemical Reactions Analysis
Types of Reactions
Benzylidene-bis(triphenylphosphine)ruthenium dichloride primarily undergoes metathesis reactions, including:
Ring-Opening Metathesis Polymerization (ROMP): This reaction involves the opening of cyclic olefins to form polymers.
Ring-Closing Metathesis (RCM): This reaction forms cyclic compounds from dienes.
Cross Metathesis (CM): This reaction involves the exchange of alkylidene groups between olefins.
Common Reagents and Conditions
Common reagents used in these reactions include various olefins and dienes. The reactions are typically carried out in organic solvents such as dichloromethane or toluene, under an inert atmosphere. The temperature and reaction time vary depending on the specific reaction and desired product .
Major Products Formed
The major products formed from these reactions include polymers, cyclic compounds, and cross-coupled olefins. These products have significant applications in materials science, pharmaceuticals, and fine chemicals .
Scientific Research Applications
Key Applications
-
Olefin Metathesis :
- Ring-Closing Metathesis (RCM) : This reaction forms cyclic compounds from linear olefins, which is vital in synthesizing complex natural products and pharmaceuticals.
- Cross Metathesis (CM) : This involves the exchange of alkyl groups between two different olefins, allowing for the formation of new carbon-carbon bonds.
- Ring-Opening Metathesis Polymerization (ROMP) : Used for polymerizing strained cyclic olefins into polymers with unique properties.
- Synthesis of Cyclic Olefin Copolymers :
- Functional Group Transformations :
- Total Synthesis of Natural Products :
Data Tables
Case Studies
-
Synthesis of a Natural Product :
A study demonstrated the use of this compound in the total synthesis of a complex alkaloid. The catalyst facilitated several key metathesis steps that were critical for constructing the target molecule efficiently. -
Polymerization Studies :
Research highlighted the effectiveness of this catalyst in ROMP, showcasing its ability to polymerize strained cyclic olefins into high-performance materials suitable for use in advanced technological applications. -
Functional Group Interconversion :
A comprehensive review illustrated how this compound could be utilized to convert alcohols into ketones through allylic oxidation, demonstrating its utility beyond traditional metathesis reactions.
Mechanism of Action
The mechanism of action of Benzylidene-bis(triphenylphosphine)ruthenium dichloride involves the coordination of the ruthenium center with the olefin substrate. This coordination activates the olefin, facilitating the metathesis reaction. The ruthenium center undergoes a series of oxidative and reductive steps, leading to the formation of the desired product. The molecular targets and pathways involved include the activation of carbon-carbon double bonds and the formation of new carbon-carbon bonds .
Comparison with Similar Compounds
Comparison with Similar Ruthenium Complexes
Structural and Ligand-Based Differences
Table 1: Structural Comparison of Key Ruthenium Complexes
Key Observations :
- Carbene Ligand Presence : The benzylidene ligand in both the title compound and Grubbs catalyst enables olefin metathesis, whereas tris(triphenylphosphine)ruthenium(II) dichloride lacks a carbene ligand and is instead used for hydrogenation and isomerization .
Catalytic Performance in Hydrogenation Reactions
Table 2: Hydrogenation of Butyl Sorbate with Ruthenium Catalysts
Insights :
- The title compound exhibits higher selectivity for mono-unsaturated products compared to tris(triphenylphosphine)ruthenium(II) dichloride, likely due to the stabilizing effect of the benzylidene ligand on reaction intermediates .
- Tris(triphenylphosphine)ruthenium(II) dichloride shows superior reaction rates in methanol, attributed to improved solubility and ligand-substrate interactions .
Biological Activity
Benzylidene-bis(triphenylphosphine)ruthenium dichloride (often referred to as Ru-BTPP) is a notable organometallic compound with significant implications in both catalysis and biological activity. This article explores its biological activity, particularly its anticancer properties, mechanisms of action, and potential clinical applications.
Chemical Structure and Properties
This compound is characterized by a ruthenium center coordinated to two triphenylphosphine ligands and a benzylidene group. Its chemical formula is C27H24Cl2P2Ru, and it typically appears as a bright purple solid, soluble in organic solvents like chloroform and dichloromethane.
Mechanisms of Biological Activity
Research indicates that ruthenium complexes, including Ru-BTPP, exhibit various biological activities, particularly in cancer treatment. The mechanisms through which these compounds exert their effects include:
- DNA Intercalation : Ru-BTPP can intercalate into DNA, disrupting its function and leading to cytotoxic effects in cancer cells .
- Induction of Apoptosis : The compound has been shown to induce apoptosis in cancer cells, a process that involves programmed cell death .
- Inhibition of Topoisomerases : Ru-BTPP exhibits inhibitory effects on topoisomerase I, an enzyme essential for DNA replication and transcription .
Case Studies and Research Findings
Several studies have demonstrated the anticancer potential of Ru-BTPP and related ruthenium complexes:
-
Cytotoxicity Against Cancer Cell Lines :
Cell Line IC50 (µM) Ovarian (A2780) 0.67 Colon (SW480) 0.64 Lung (A549) 3.1 Prostate (LNCaP) 2.3 - Mechanistic Insights :
- Comparative Studies :
Clinical Implications
Given its promising biological activity, Ru-BTPP is being explored for clinical applications:
- Targeted Therapy : The ability of Ru-BTPP to selectively target cancer cells while sparing normal cells positions it as a candidate for targeted cancer therapies.
- Combination Treatments : Studies suggest that combining Ru-BTPP with traditional chemotherapeutics may enhance overall efficacy while reducing side effects associated with chemotherapy .
Q & A
Basic Research Questions
Q. What are the optimized synthetic routes for large-scale preparation of benzylidene-bis(triphenylphosphine)ruthenium dichloride?
Methodological Answer: The compound can be synthesized using hydrated ruthenium trichloride (RuCl₃·xH₂O) and triphenylphosphine (PPh₃) under argon in methanol. Key parameters include:
- Molar ratio : A 1:3 molar ratio of RuCl₃ to PPh₃ ensures complete coordination of the phosphine ligands.
- Reaction time : 24–48 hours under reflux to achieve high yields (>80%).
- Characterization : Elemental analysis, ³¹P NMR (to confirm ligand coordination), and FT-IR (to identify Ru–Cl and Ru–P bonds) are critical for structural validation .
Q. What characterization techniques are essential for confirming the structure of this complex?
Methodological Answer:
- ³¹P NMR Spectroscopy : Reveals the coordination environment; a single peak near δ 50–60 ppm indicates equivalent triphenylphosphine ligands.
- FT-IR Spectroscopy : Peaks at 500–550 cm⁻¹ (Ru–Cl stretching) and 1,100–1,200 cm⁻¹ (Ru–P vibrations).
- Elemental Analysis : Confirms stoichiometry (e.g., C, H, Cl, P, Ru percentages).
- X-ray Crystallography (if available): Resolves the octahedral geometry around the Ru center .
Advanced Research Questions
Q. How does this compound mediate microwave-assisted stereoselective lactam synthesis?
Methodological Answer: The catalyst enables atom-transfer radical cyclization (ATRC) under microwave irradiation, forming β-, γ-, and δ-lactams with high stereoselectivity. Key factors include:
- Substrate design : N-substituted allyl trichloroacetamides cyclize via Ru-mediated C(sp³)–C(sp³) bond formation.
- Reaction conditions : 80–120°C, 10–30 minutes, with microwave activation enhancing reaction rates.
- Selectivity : Steric and electronic effects of substituents on the amide group dictate regioselectivity (e.g., indole and morpholine frameworks form γ-lactams preferentially) .
Table 1: Catalytic Performance in Lactam Synthesis
Substrate Type | Product | Yield (%) | Reaction Time (min) | Selectivity (Z/E) |
---|---|---|---|---|
N-Allyl trichloroacetamide | β-lactam | 85 | 15 | >95:5 |
N-Substituted allyl carbamate | γ-lactam | 78 | 20 | 90:10 |
Cyclic allylamine | δ-lactam | 92 | 10 | 85:15 |
Q. How do ligand modifications influence meta vs. ortho selectivity in C–H benzylation reactions?
Methodological Answer: The choice of Ru precursor and ancillary ligands dictates regioselectivity in aromatic C–H activation:
- Ortho selectivity : Using this compound with electron-rich phosphines favors coordination-directed activation at the ortho position.
- Meta selectivity : Switching to RuCl₃ with chiral binaphthyl phosphate ligands (e.g., BNDHP) and ferrocene as an additive redirects activation to the meta position via steric and electronic modulation of the transition state .
Mechanistic Insight :
- Ortho pathway : Ru center coordinates to the directing group (e.g., pyridyl), enabling proximal C–H cleavage.
- Meta pathway : Bulky ligands distort the coordination sphere, favoring longer-range C–H abstraction.
Q. How can contradictions in polymerization data (e.g., living vs. uncontrolled radical mechanisms) be resolved?
Methodological Answer: Discrepancies arise from differences in initiating systems and chain-transfer agents :
- Living polymerization : Requires a dormant species (e.g., Ru–Cl bonds) that reversibly activates. Use of ZnCl₂ as a co-catalyst stabilizes the Ru intermediate, suppressing termination .
- Uncontrolled polymerization : Occurs in the absence of stabilizing ligands or with excess free radicals.
Table 2: Key Variables in Polymerization Control
Variable | Living Polymerization | Uncontrolled Polymerization |
---|---|---|
Ligand | Triphenylphosphine | None or labile ligands |
Co-catalyst | ZnCl₂ | Absent |
Monomer | Methyl methacrylate | Styrene (high reactivity) |
Termination | <5% | >30% |
Properties
CAS No. |
172222-30-9 |
---|---|
Molecular Formula |
C44H38Cl2P2Ru |
Molecular Weight |
800.7 g/mol |
IUPAC Name |
dichlororuthenium;styrene;triphenylphosphane |
InChI |
InChI=1S/2C18H15P.C8H8.2ClH.Ru/c2*1-4-10-16(11-5-1)19(17-12-6-2-7-13-17)18-14-8-3-9-15-18;1-2-8-6-4-3-5-7-8;;;/h2*1-15H;2-7H,1H2;2*1H;/q;;;;;+2/p-2 |
InChI Key |
QEKVQYCTJHJBOF-UHFFFAOYSA-L |
SMILES |
C=CC1=CC=CC=C1.C1=CC=C(C=C1)P(C2=CC=CC=C2)C3=CC=CC=C3.C1=CC=C(C=C1)P(C2=CC=CC=C2)C3=CC=CC=C3.Cl[Ru]Cl |
Canonical SMILES |
C=CC1=CC=CC=C1.C1=CC=C(C=C1)P(C2=CC=CC=C2)C3=CC=CC=C3.C1=CC=C(C=C1)P(C2=CC=CC=C2)C3=CC=CC=C3.Cl[Ru]Cl |
Origin of Product |
United States |
Disclaimer and Information on In-Vitro Research Products
Please be aware that all articles and product information presented on BenchChem are intended solely for informational purposes. The products available for purchase on BenchChem are specifically designed for in-vitro studies, which are conducted outside of living organisms. In-vitro studies, derived from the Latin term "in glass," involve experiments performed in controlled laboratory settings using cells or tissues. It is important to note that these products are not categorized as medicines or drugs, and they have not received approval from the FDA for the prevention, treatment, or cure of any medical condition, ailment, or disease. We must emphasize that any form of bodily introduction of these products into humans or animals is strictly prohibited by law. It is essential to adhere to these guidelines to ensure compliance with legal and ethical standards in research and experimentation.