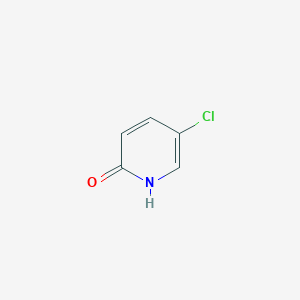
5-Chloro-2-hydroxypyridine
Overview
Description
5-Chloro-2-hydroxypyridine: is an organic compound with the molecular formula C5H4ClNO . It is a derivative of pyridine, where the hydrogen atom at the 5-position is replaced by a chlorine atom, and the hydrogen atom at the 2-position is replaced by a hydroxyl group. This compound is known for its applications in various fields, including chemistry, biology, and medicine.
Scientific Research Applications
Chemistry:
Ligand in Coordination Chemistry:
Biology and Medicine:
Enzyme Inhibition Studies: It has been used to study the effect of dicumarol on xanthine dehydrogenase and the mechanism of mitomycin C bioreduction by xanthine dehydrogenase.
Industry:
Intermediate in Synthesis: This compound serves as an intermediate in the synthesis of various pharmaceuticals and agrochemicals.
Mechanism of Action
Target of Action
5-Chloro-2-hydroxypyridine, also known as 5-Chloro-2-pyridinol, primarily targets xanthine dehydrogenase . Xanthine dehydrogenase is an enzyme involved in the metabolic breakdown of purines in the body, which are vital components of DNA, RNA, and ATP.
Mode of Action
This compound acts as a donor ligand . It exists in a zwitterionic form, i.e., 5-chloropyridinium-2-olate, in copper complexes
Biochemical Pathways
The primary biochemical pathway affected by this compound is the purine degradation pathway . By interacting with xanthine dehydrogenase, it can influence the breakdown of purines, potentially affecting the levels of uric acid, a product of purine metabolism.
Pharmacokinetics
Given its molecular weight of 12954 , it is likely to have good bioavailability
Result of Action
This compound has been used to study the effect of dicumarol on xanthine dehydrogenase . It has also been used to investigate the mechanism of mitomycin C bioreduction by xanthine dehydrogenase . The specific molecular and cellular effects of this compound’s action depend on the context of its use and require further investigation.
Safety and Hazards
5-Chloro-2-hydroxypyridine causes skin irritation and serious eye irritation. It is recommended to avoid breathing mist, gas, or vapors, avoid contacting with skin and eye, use personal protective equipment, wear chemical impermeable gloves, ensure adequate ventilation, remove all sources of ignition, evacuate personnel to safe areas, and keep people away from and upwind of spill/leak .
Future Directions
Biochemical Analysis
Biochemical Properties
5-Chloro-2-hydroxypyridine acts as a donor ligand and exists in a zwitterionic form, i.e., 5-chloropyridinium-2-olate, in copper complexes . It has been used to study the effect of dicumarol on xanthine dehydrogenase and the mechanism of mitomycin C bioreduction by xanthine dehydrogenase
Molecular Mechanism
It is known to act as a donor ligand in copper complexes , suggesting it may interact with biomolecules through binding interactions
Preparation Methods
Synthetic Routes and Reaction Conditions:
Chlorination of 2-hydroxypyridine: One common method involves the chlorination of 2-hydroxypyridine using chlorinating agents such as phosphorus oxychloride or phosphorus pentachloride.
N-chlorosuccinimide Method: Another method involves the reaction of 2,6-dimethylpyridine-4-phenol with N-chlorosuccinimide in the presence of a solvent like dimethylformamide.
Industrial Production Methods:
- Industrial production methods often involve large-scale chlorination processes using efficient chlorinating agents and optimized reaction conditions to ensure high yield and purity of the product.
Chemical Reactions Analysis
Types of Reactions:
Substitution Reactions: 5-Chloro-2-hydroxypyridine can undergo nucleophilic substitution reactions where the chlorine atom is replaced by other nucleophiles.
Complex Formation: This compound can act as a donor ligand and form complexes with metals such as copper.
Common Reagents and Conditions:
Nucleophilic Substitution: Potassium carbonate in methanol is a common reagent for substitution reactions.
Complex Formation: Copper salts are commonly used for forming metal complexes.
Major Products:
2-Hydroxy-5-methoxypyridine: Formed from nucleophilic substitution reactions.
Copper Complexes: Formed from complexation reactions with copper salts.
Comparison with Similar Compounds
6-Chloro-2-hydroxypyridine: Similar in structure but with the chlorine atom at the 6-position instead of the 5-position.
2-Chloro-3-hydroxypyridine: Another similar compound with the chlorine atom at the 2-position and the hydroxyl group at the 3-position.
5-Bromo-2-hydroxypyridine: Similar compound where the chlorine atom is replaced by a bromine atom.
Uniqueness:
Position of Substituents: The unique positioning of the chlorine and hydroxyl groups in 5-Chloro-2-hydroxypyridine gives it distinct chemical properties and reactivity compared to its analogs.
Reactivity: The presence of the chlorine atom at the 5-position makes it more reactive in nucleophilic substitution reactions compared to other isomers.
Properties
IUPAC Name |
5-chloro-1H-pyridin-2-one | |
---|---|---|
Source | PubChem | |
URL | https://pubchem.ncbi.nlm.nih.gov | |
Description | Data deposited in or computed by PubChem | |
InChI |
InChI=1S/C5H4ClNO/c6-4-1-2-5(8)7-3-4/h1-3H,(H,7,8) | |
Source | PubChem | |
URL | https://pubchem.ncbi.nlm.nih.gov | |
Description | Data deposited in or computed by PubChem | |
InChI Key |
SZFUWUOHDRMCKD-UHFFFAOYSA-N | |
Source | PubChem | |
URL | https://pubchem.ncbi.nlm.nih.gov | |
Description | Data deposited in or computed by PubChem | |
Canonical SMILES |
C1=CC(=O)NC=C1Cl | |
Source | PubChem | |
URL | https://pubchem.ncbi.nlm.nih.gov | |
Description | Data deposited in or computed by PubChem | |
Molecular Formula |
C5H4ClNO | |
Source | PubChem | |
URL | https://pubchem.ncbi.nlm.nih.gov | |
Description | Data deposited in or computed by PubChem | |
DSSTOX Substance ID |
DTXSID1022215 | |
Record name | 5-Chloro-2-pyridinol | |
Source | EPA DSSTox | |
URL | https://comptox.epa.gov/dashboard/DTXSID1022215 | |
Description | DSSTox provides a high quality public chemistry resource for supporting improved predictive toxicology. | |
Molecular Weight |
129.54 g/mol | |
Source | PubChem | |
URL | https://pubchem.ncbi.nlm.nih.gov | |
Description | Data deposited in or computed by PubChem | |
CAS No. |
4214-79-3 | |
Record name | 5-Chloro-2(1H)-pyridinone | |
Source | CAS Common Chemistry | |
URL | https://commonchemistry.cas.org/detail?cas_rn=4214-79-3 | |
Description | CAS Common Chemistry is an open community resource for accessing chemical information. Nearly 500,000 chemical substances from CAS REGISTRY cover areas of community interest, including common and frequently regulated chemicals, and those relevant to high school and undergraduate chemistry classes. This chemical information, curated by our expert scientists, is provided in alignment with our mission as a division of the American Chemical Society. | |
Explanation | The data from CAS Common Chemistry is provided under a CC-BY-NC 4.0 license, unless otherwise stated. | |
Record name | 5-Chloro-2-pyridinol | |
Source | ChemIDplus | |
URL | https://pubchem.ncbi.nlm.nih.gov/substance/?source=chemidplus&sourceid=0004214793 | |
Description | ChemIDplus is a free, web search system that provides access to the structure and nomenclature authority files used for the identification of chemical substances cited in National Library of Medicine (NLM) databases, including the TOXNET system. | |
Record name | 5-Chloro-2-pyridinol | |
Source | EPA DSSTox | |
URL | https://comptox.epa.gov/dashboard/DTXSID1022215 | |
Description | DSSTox provides a high quality public chemistry resource for supporting improved predictive toxicology. | |
Record name | 5-chloropyridin-2-ol | |
Source | European Chemicals Agency (ECHA) | |
URL | https://echa.europa.eu/substance-information/-/substanceinfo/100.021.952 | |
Description | The European Chemicals Agency (ECHA) is an agency of the European Union which is the driving force among regulatory authorities in implementing the EU's groundbreaking chemicals legislation for the benefit of human health and the environment as well as for innovation and competitiveness. | |
Explanation | Use of the information, documents and data from the ECHA website is subject to the terms and conditions of this Legal Notice, and subject to other binding limitations provided for under applicable law, the information, documents and data made available on the ECHA website may be reproduced, distributed and/or used, totally or in part, for non-commercial purposes provided that ECHA is acknowledged as the source: "Source: European Chemicals Agency, http://echa.europa.eu/". Such acknowledgement must be included in each copy of the material. ECHA permits and encourages organisations and individuals to create links to the ECHA website under the following cumulative conditions: Links can only be made to webpages that provide a link to the Legal Notice page. | |
Record name | 5-CHLORO-2-PYRIDINOL | |
Source | FDA Global Substance Registration System (GSRS) | |
URL | https://gsrs.ncats.nih.gov/ginas/app/beta/substances/R7A6F5D3KY | |
Description | The FDA Global Substance Registration System (GSRS) enables the efficient and accurate exchange of information on what substances are in regulated products. Instead of relying on names, which vary across regulatory domains, countries, and regions, the GSRS knowledge base makes it possible for substances to be defined by standardized, scientific descriptions. | |
Explanation | Unless otherwise noted, the contents of the FDA website (www.fda.gov), both text and graphics, are not copyrighted. They are in the public domain and may be republished, reprinted and otherwise used freely by anyone without the need to obtain permission from FDA. Credit to the U.S. Food and Drug Administration as the source is appreciated but not required. | |
Synthesis routes and methods
Procedure details
Retrosynthesis Analysis
AI-Powered Synthesis Planning: Our tool employs the Template_relevance Pistachio, Template_relevance Bkms_metabolic, Template_relevance Pistachio_ringbreaker, Template_relevance Reaxys, Template_relevance Reaxys_biocatalysis model, leveraging a vast database of chemical reactions to predict feasible synthetic routes.
One-Step Synthesis Focus: Specifically designed for one-step synthesis, it provides concise and direct routes for your target compounds, streamlining the synthesis process.
Accurate Predictions: Utilizing the extensive PISTACHIO, BKMS_METABOLIC, PISTACHIO_RINGBREAKER, REAXYS, REAXYS_BIOCATALYSIS database, our tool offers high-accuracy predictions, reflecting the latest in chemical research and data.
Strategy Settings
Precursor scoring | Relevance Heuristic |
---|---|
Min. plausibility | 0.01 |
Model | Template_relevance |
Template Set | Pistachio/Bkms_metabolic/Pistachio_ringbreaker/Reaxys/Reaxys_biocatalysis |
Top-N result to add to graph | 6 |
Feasible Synthetic Routes
Q1: Has 5-Chloro-2-hydroxypyridine been utilized in material science applications?
A: Yes, this compound derivatives have shown promise in perovskite solar cell technology. Studies reveal that incorporating a derivative with an amino group (–NH2) at a specific position on the this compound molecule can enhance the performance and durability of perovskite solar cells. [] This is attributed to the molecule's ability to passivate defects within the perovskite structure, improving charge carrier dynamics. []
Q2: How does the structure of this compound relate to its coordination chemistry?
A: this compound can act as a ligand in coordination complexes. In the complex catena-Poly[[[diaquabis(5-chloropyridinium-2-olato-κO)copper(II)]-μ-pyrazine-κ2N:N’] diperchlorate], this compound exists in its zwitterionic form, 5-chloropyridinium-2-olate. [, ] In this form, it coordinates to copper (II) ions via the oxygen atom, forming a chain structure bridged by pyrazine ligands. [, ] This highlights the versatility of this compound in adopting different forms depending on the chemical environment.
Q3: What spectroscopic techniques have been employed to characterize this compound?
A: Rotational spectroscopy has been successfully used to study the gas-phase tautomeric preferences of this compound. [] This technique, combined with a full quadrupolar hyperfine analysis due to the chlorine and nitrogen nuclei, allows for the unambiguous identification of different tautomers. [] Additionally, vibrational spectroscopic techniques like FT-IR and FT-Raman spectroscopy are likely used to characterize the molecule, although specific data from the provided research is not available. []
Q4: Are there computational studies on this compound and its derivatives?
A: Density functional theory (DFT) calculations have been employed to investigate the electronic properties and behavior of this compound derivatives. [] Specifically, DFT studies were used to analyze the impact of different substituents on the molecule's dipole moment and electron density distribution. [] This information is valuable for understanding its interaction with the perovskite material in solar cell applications. [] Molecular docking studies have also been explored, potentially to evaluate interactions with biomolecules like insulin. []
Disclaimer and Information on In-Vitro Research Products
Please be aware that all articles and product information presented on BenchChem are intended solely for informational purposes. The products available for purchase on BenchChem are specifically designed for in-vitro studies, which are conducted outside of living organisms. In-vitro studies, derived from the Latin term "in glass," involve experiments performed in controlled laboratory settings using cells or tissues. It is important to note that these products are not categorized as medicines or drugs, and they have not received approval from the FDA for the prevention, treatment, or cure of any medical condition, ailment, or disease. We must emphasize that any form of bodily introduction of these products into humans or animals is strictly prohibited by law. It is essential to adhere to these guidelines to ensure compliance with legal and ethical standards in research and experimentation.