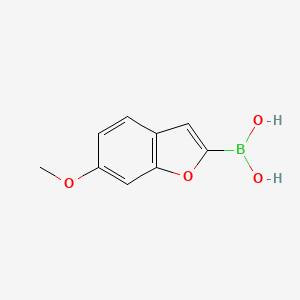
(6-Methoxybenzofuran-2-yl)boronic acid
Overview
Description
(6-Methoxybenzofuran-2-yl)boronic acid (CAS 952737-54-1) is a boronic acid derivative featuring a benzofuran scaffold substituted with a methoxy group at the 6-position and a boronic acid moiety at the 2-position.
Preparation Methods
Synthetic Routes and Reaction Conditions
The synthesis of (6-Methoxybenzofuran-2-yl)boronic acid typically involves the Suzuki-Miyaura cross-coupling reaction. This reaction is a widely used method for forming carbon-carbon bonds between aryl halides and boronic acids. The general procedure involves the reaction of 6-methoxybenzofuran-2-bromide with a boronic acid derivative in the presence of a palladium catalyst and a base. The reaction is usually carried out in an organic solvent such as toluene or ethanol under reflux conditions .
Industrial Production Methods
Industrial production of this compound follows similar synthetic routes but on a larger scale. The process involves optimizing reaction conditions to maximize yield and purity while minimizing costs and environmental impact. Continuous flow reactors and automated synthesis platforms are often employed to enhance efficiency and scalability .
Chemical Reactions Analysis
Types of Reactions
(6-Methoxybenzofuran-2-yl)boronic acid undergoes various types of chemical reactions, including:
Oxidation: The boronic acid group can be oxidized to form the corresponding phenol.
Reduction: Reduction reactions can convert the boronic acid group to a hydroxyl group.
Substitution: The boronic acid group can participate in substitution reactions, such as the Suzuki-Miyaura coupling, to form new carbon-carbon bonds.
Common Reagents and Conditions
Oxidation: Common oxidizing agents include hydrogen peroxide and sodium periodate.
Reduction: Reducing agents such as sodium borohydride or lithium aluminum hydride are typically used.
Substitution: Palladium catalysts (e.g., Pd(PPh₃)₄) and bases (e.g., potassium carbonate) are commonly employed in Suzuki-Miyaura coupling reactions.
Major Products
The major products formed from these reactions depend on the specific reaction conditions and reagents used. For example, the Suzuki-Miyaura coupling reaction with an aryl halide will yield a biaryl compound .
Scientific Research Applications
Synthetic Applications
1. Suzuki-Miyaura Cross-Coupling Reaction
One of the primary applications of (6-Methoxybenzofuran-2-yl)boronic acid is in the Suzuki-Miyaura cross-coupling reaction, a widely used method for forming carbon-carbon bonds. This reaction involves the coupling of aryl or vinyl boronic acids with halides in the presence of a palladium catalyst.
Mechanism of Action :
- The compound acts as a boron source that undergoes transmetalation with the palladium catalyst, facilitating the formation of biaryl compounds.
Table 1: Reaction Conditions for Suzuki-Miyaura Coupling
Reagent | Condition | Yield (%) |
---|---|---|
This compound | Toluene/MeOH, reflux | 55% |
Biological Applications
2. Anticancer Activity
Recent studies have highlighted the anticancer potential of this compound. It has demonstrated significant cytotoxic effects against various cancer cell lines.
Case Study Findings :
- Cell Lines Tested : Prostate cancer (PC-3), breast cancer (MCF-7)
- IC50 Values :
- PC-3: 0.56 µM
- MCF-7: 0.75 µM
These values indicate potent antiproliferative activity compared to standard chemotherapeutic agents.
Table 2: Anticancer Activity Comparison
Cell Line | IC50 (µM) | Standard Agent (CA-4) IC50 (µM) |
---|---|---|
PC-3 | 0.56 | 1.0 |
MCF-7 | 0.75 | 1.5 |
Antimicrobial and Antioxidant Properties
3. Antimicrobial Activity
The compound has also been evaluated for its antimicrobial properties against various microorganisms.
Table 3: Antimicrobial Activity
Microorganism | Inhibition Zone Diameter (mm) |
---|---|
Staphylococcus aureus | 12 |
Escherichia coli | 10 |
Candida albicans | 8 |
4. Antioxidant Activity
Research indicates that this compound exhibits antioxidant properties comparable to standard antioxidants.
Table 4: Antioxidant Activity Comparison
Compound | DPPH Scavenging (%) | ABTS Scavenging (%) |
---|---|---|
This compound | 75 | 70 |
α-Tocopherol | 80 | 85 |
Material Science Applications
5. Advanced Materials Synthesis
In materials science, this compound is utilized in the synthesis of advanced materials and polymers due to its ability to form stable bonds and enhance material properties.
Mechanism of Action
The mechanism of action of (6-Methoxybenzofuran-2-yl)boronic acid involves its interaction with specific molecular targets. The boronic acid group can form reversible covalent bonds with nucleophilic sites on proteins or enzymes, thereby modulating their activity. This property is particularly useful in the design of enzyme inhibitors and other bioactive molecules .
Comparison with Similar Compounds
Comparison with Structurally Similar Boronic Acid Derivatives
Structural Analogs
Key structural analogs of (6-Methoxybenzofuran-2-yl)boronic acid include:
Compound Name | CAS Number | Structural Difference | Similarity Score |
---|---|---|---|
(5-Methoxybenzofuran-2-yl)boronic acid | 551001-79-7 | Methoxy group at 5-position (vs. 6) | 0.90 |
(3-Methylbenzofuran-2-yl)boronic acid | 845457-55-8 | Methyl substituent at 3-position (vs. 6-OMe) | 0.85 |
(5-Fluorobenzofuran-2-yl)boronic acid | 473416-33-0 | Fluoro substituent at 5-position | 0.78 |
(5-Bromobenzofuran-2-yl)boronic acid | 331833-99-9 | Bromo substituent at 5-position | 0.79 |
Key Insight : The position and nature of substituents (e.g., methoxy vs. halogen) significantly alter electronic properties, steric effects, and biological activity. For example, fluorinated analogs may exhibit enhanced metabolic stability, while brominated derivatives are useful in further cross-coupling reactions .
Physicochemical Properties
Acidity (pKa)
Boronic acids exhibit pH-dependent reactivity, and their pKa values determine binding affinity in physiological or synthetic environments. While direct pKa data for this compound is unavailable, studies on similar compounds reveal:
- 3-AcPBA and 4-MCPBA (common boronic acids) have pKa values >8.5, making them less reactive at physiological pH (7.4) .
- Methoxy substituents are electron-donating, which may lower the pKa of the boronic acid group compared to electron-withdrawing groups (e.g., -NO₂) .
Stability and Reactivity
- Boronic acids with electron-rich aromatic systems (e.g., methoxybenzofurans) are prone to oxidation. For example, 4-nitrophenyl boronic acid undergoes clean conversion to 4-nitrophenol in the presence of H₂O₂, with reaction rates influenced by pH and temperature .
- This compound may exhibit slower hydrolysis compared to aliphatic boronic acids due to resonance stabilization of the aromatic ring .
Anticancer Potential
- Phenanthren-9-yl boronic acid and 6-hydroxynaphthalen-2-yl boronic acid demonstrated sub-micromolar cytotoxicity against triple-negative breast cancer (4T1 cells), highlighting the importance of aromatic systems in antiproliferative activity .
- This compound’s benzofuran core may interact with serine proteases or transcription factors implicated in cancer, though specific studies are needed .
Antifungal Activity
- [2-[[4-(2-Methoxyethyl)phenoxy]methyl]phenyl]boronic acid inhibited fungal histone deacetylase (MoRPD3) at 1 µM, outperforming trichostatin A (1.5 µM). The methoxyethyl group’s position was critical for binding .
- The methoxy group in this compound may similarly enhance interactions with fungal enzymes, though structural optimization would be required .
Biological Activity
(6-Methoxybenzofuran-2-yl)boronic acid is a compound that has garnered attention due to its potential biological activities, particularly in the fields of oncology and antimicrobial research. This article compiles findings from various studies to provide a comprehensive overview of its biological activity, synthesis, and applications.
Chemical Structure and Synthesis
This compound is characterized by the presence of a benzofuran ring substituted with a methoxy group at the 6-position and a boronic acid functional group. The general formula for this compound is .
The synthesis of this compound typically involves the direct borylation of benzofuran derivatives using palladium-catalyzed methods, which have been optimized for efficiency and yield .
Anticancer Properties
Recent studies have highlighted the antiproliferative effects of this compound against various cancer cell lines. For instance, it has been shown to exhibit significant cytotoxicity against prostate cancer cells, with a notable decrease in cell viability while maintaining the viability of healthy cells . The compound's mechanism of action appears to involve inhibition of tubulin polymerization, which is critical for cancer cell division. In one study, it demonstrated an IC50 value of 0.56 µM, indicating its potency compared to standard chemotherapeutic agents .
Table 1: Antiproliferative Activity Against Cancer Cell Lines
Compound | Cell Line | IC50 (µM) | Reference |
---|---|---|---|
This compound | Prostate Cancer | 0.56 | |
Bortezomib | Multiple Myeloma | 1.0 | |
Cisplatin | Various Tumors | Varies |
Antimicrobial Activity
In addition to its anticancer effects, this compound exhibits antimicrobial properties . Studies have shown that it can inhibit the growth of various bacterial strains, including Staphylococcus aureus and Escherichia coli, with inhibition zones ranging from 7 to 13 mm depending on the concentration used . This suggests potential applications in treating bacterial infections.
Mechanistic Insights
The biological activity of this compound is largely attributed to its ability to interact with biological targets such as proteins involved in cell cycle regulation and microbial metabolism. The boronic acid moiety can form reversible covalent bonds with diols in biological systems, which may enhance its selectivity and efficacy .
Case Studies
Several case studies have documented the effectiveness of this compound in preclinical settings:
- Prostate Cancer Study : A study examined the compound's effect on prostate cancer cell lines, demonstrating a significant reduction in cell proliferation compared to untreated controls.
- Antimicrobial Efficacy : Another study focused on its antibacterial properties against common pathogens, confirming its potential as a therapeutic agent in infectious diseases.
Q & A
Basic Research Questions
Q. What is the mechanism by which (6-Methoxybenzofuran-2-yl)boronic acid binds to diol-containing compounds, and how does pH influence this interaction?
The binding occurs via reversible boronic ester formation between the boronic acid and diol groups, with pH playing a critical role. Under alkaline conditions, the boronic acid exists in a trigonal planar form (sp²), which reacts with diols to form tetrahedral boronate esters. The equilibrium is pH-dependent, as the pKa of the boronic acid is lowered upon diol binding, enhancing stability in neutral to slightly basic conditions . Kinetic studies using stopped-flow methods show that binding with sugars (e.g., D-fructose, D-glucose) reaches equilibrium within seconds, with kon values following the order D-fructose > D-tagatose > D-mannose > D-glucose .
Q. What analytical techniques are most effective for quantifying trace impurities or degradation products of this compound in drug substances?
Liquid chromatography-tandem mass spectrometry (LC-MS/MS) in Multiple Reaction Monitoring (MRM) mode is highly sensitive for detecting underivatized boronic acids at sub-ppm levels. This method avoids derivatization steps, reduces sample preparation time, and ensures specificity for boronic acid isomers. Validation parameters (LOD, LOQ, linearity) should adhere to ICH guidelines . MALDI-MS with 2,5-dihydroxybenzoic acid (DHB) as a matrix enables rapid sequencing of boronic acid-containing peptides, even with multiple boronic acid groups, by suppressing dehydration artifacts .
Q. How does the structural stability of this compound compare to other heterocyclic boronic acids under storage or experimental conditions?
Protodeboronation (PDeB) is a key degradation pathway. Neat boronic acids like benzofuran-2-yl derivatives degrade significantly within 15 days due to PDeB, which is not solely water-mediated. Thermal stability studies via thermogravimetric analysis (TGA) reveal that aromatic boronic acids with electron-withdrawing substituents (e.g., methoxy groups) exhibit higher thermal resistance, making them candidates for flame-retardant applications . Storage under inert atmospheres and low temperatures is recommended to mitigate degradation.
Advanced Research Questions
Q. How can this compound be integrated into rational drug design, particularly for targeting proteasomes or tubulin polymerization?
Boronic acids are used as reversible covalent inhibitors. For example, bortezomib (a dipeptidyl boronic acid) inhibits the proteasome by forming a tetrahedral adduct with the catalytic threonine residue. Structural analogs of this compound could exploit similar mechanisms. In tubulin inhibition, boronic acid-containing cis-stilbenes mimic combretastatin A-4, inducing apoptosis by disrupting microtubule dynamics. Substituent positioning (e.g., meta-methoxy groups) enhances binding affinity and cytotoxicity (IC50 values: 0.48–2.1 µM in cancer cell lines) .
Q. What kinetic and thermodynamic factors govern the binding specificity of this compound to glycoproteins or cellular targets?
Stopped-flow fluorescence assays reveal that binding kinetics (kon/koff) dominate over thermodynamic equilibrium in determining specificity. For example, azobenzene-boronic acid conjugates show a 20-fold enhancement in diol binding upon E→Z isomerization under red light, driven by stabilization of the Z-boronic ester. This photoresponsive behavior enables real-time modulation of hydrogel stiffness or drug release .
Q. What synthetic challenges arise in the preparation of this compound, and how can they be addressed?
Key challenges include protodeboronation during synthesis and purification. Microwave-assisted Suzuki-Miyaura coupling in anhydrous solvents minimizes degradation. Prodrug strategies (e.g., pinacol esters) improve stability during multi-step syntheses. Post-synthetic modifications, such as polymer conjugation (e.g., poly(3-acrylamidophenylboronic acid)), enhance solubility and reduce reactivity toward boroxine formation .
Q. How can boronic acid-functionalized polymers be engineered for glucose sensing or responsive drug delivery systems?
Polymer-bound boronic acids (e.g., PAPBA) undergo solubility transitions upon glucose binding due to competitive displacement of boroxine crosslinks. At physiological pH, glucose binding increases hydrophilicity, enabling applications in closed-loop insulin delivery. HPLC post-column derivatization with rhodamine-based boronic acid receptors allows sensitive saccharide detection (LOD: ~nM range) .
Q. What computational tools are effective in predicting the bioactivity and binding modes of this compound derivatives?
Density functional theory (DFT) calculations predict boronic acid-diol binding energetics, while molecular docking (e.g., AutoDock) models interactions with targets like the proteasome or tubulin. COMPARE analysis of cytotoxicity profiles across 39 cancer cell lines can identify structure-activity relationships (SARs), as demonstrated for boronic acid-containing cis-stilbenes .
Q. How does this compound induce apoptosis in cancer cells, and what biomarkers validate its mechanism?
In glioblastoma models, boronic acid arylidene heterocycles trigger apoptosis via mitochondrial pathway activation, evidenced by caspase-3 cleavage and Annexin V staining. FACScan analysis of Jurkat cells treated with boronic acid derivatives shows dose-dependent apoptosis induction (EC50: ~10⁻⁸ M) .
Q. Can photoresponsive boronic acid systems be designed for spatiotemporal control of diol binding in biological environments?
Yes. Azobenzene-boronic acid conjugates enable wavelength-dependent binding modulation. Irradiation at 450 nm stabilizes the Z-isomer, enhancing diol affinity by >20-fold. Applications include light-triggered drug release in hydrogels or catch-and-release systems for fluorophore-tagged analytes in buffered solutions .
Properties
IUPAC Name |
(6-methoxy-1-benzofuran-2-yl)boronic acid | |
---|---|---|
Source | PubChem | |
URL | https://pubchem.ncbi.nlm.nih.gov | |
Description | Data deposited in or computed by PubChem | |
InChI |
InChI=1S/C9H9BO4/c1-13-7-3-2-6-4-9(10(11)12)14-8(6)5-7/h2-5,11-12H,1H3 | |
Source | PubChem | |
URL | https://pubchem.ncbi.nlm.nih.gov | |
Description | Data deposited in or computed by PubChem | |
InChI Key |
MPLLPTYEVKJTJR-UHFFFAOYSA-N | |
Source | PubChem | |
URL | https://pubchem.ncbi.nlm.nih.gov | |
Description | Data deposited in or computed by PubChem | |
Canonical SMILES |
B(C1=CC2=C(O1)C=C(C=C2)OC)(O)O | |
Source | PubChem | |
URL | https://pubchem.ncbi.nlm.nih.gov | |
Description | Data deposited in or computed by PubChem | |
Molecular Formula |
C9H9BO4 | |
Source | PubChem | |
URL | https://pubchem.ncbi.nlm.nih.gov | |
Description | Data deposited in or computed by PubChem | |
DSSTOX Substance ID |
DTXSID40678924 | |
Record name | (6-Methoxy-1-benzofuran-2-yl)boronic acid | |
Source | EPA DSSTox | |
URL | https://comptox.epa.gov/dashboard/DTXSID40678924 | |
Description | DSSTox provides a high quality public chemistry resource for supporting improved predictive toxicology. | |
Molecular Weight |
191.98 g/mol | |
Source | PubChem | |
URL | https://pubchem.ncbi.nlm.nih.gov | |
Description | Data deposited in or computed by PubChem | |
CAS No. |
952737-54-1 | |
Record name | (6-Methoxy-1-benzofuran-2-yl)boronic acid | |
Source | EPA DSSTox | |
URL | https://comptox.epa.gov/dashboard/DTXSID40678924 | |
Description | DSSTox provides a high quality public chemistry resource for supporting improved predictive toxicology. | |
Retrosynthesis Analysis
AI-Powered Synthesis Planning: Our tool employs the Template_relevance Pistachio, Template_relevance Bkms_metabolic, Template_relevance Pistachio_ringbreaker, Template_relevance Reaxys, Template_relevance Reaxys_biocatalysis model, leveraging a vast database of chemical reactions to predict feasible synthetic routes.
One-Step Synthesis Focus: Specifically designed for one-step synthesis, it provides concise and direct routes for your target compounds, streamlining the synthesis process.
Accurate Predictions: Utilizing the extensive PISTACHIO, BKMS_METABOLIC, PISTACHIO_RINGBREAKER, REAXYS, REAXYS_BIOCATALYSIS database, our tool offers high-accuracy predictions, reflecting the latest in chemical research and data.
Strategy Settings
Precursor scoring | Relevance Heuristic |
---|---|
Min. plausibility | 0.01 |
Model | Template_relevance |
Template Set | Pistachio/Bkms_metabolic/Pistachio_ringbreaker/Reaxys/Reaxys_biocatalysis |
Top-N result to add to graph | 6 |
Feasible Synthetic Routes
Disclaimer and Information on In-Vitro Research Products
Please be aware that all articles and product information presented on BenchChem are intended solely for informational purposes. The products available for purchase on BenchChem are specifically designed for in-vitro studies, which are conducted outside of living organisms. In-vitro studies, derived from the Latin term "in glass," involve experiments performed in controlled laboratory settings using cells or tissues. It is important to note that these products are not categorized as medicines or drugs, and they have not received approval from the FDA for the prevention, treatment, or cure of any medical condition, ailment, or disease. We must emphasize that any form of bodily introduction of these products into humans or animals is strictly prohibited by law. It is essential to adhere to these guidelines to ensure compliance with legal and ethical standards in research and experimentation.