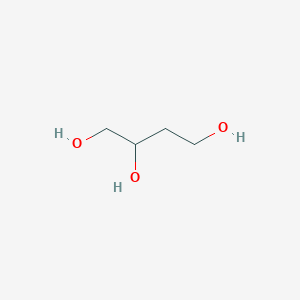
1,2,4-Butanetriol
Overview
Description
1,2,4-Butanetriol is a clear yellow to slightly brownish liquid . It is an alcohol with three hydrophilic alcoholic hydroxyl groups . It is used as a starting reagent during the synthesis of a series of quaternary ammonium lipids and in the synthesis of (-)-γ-amino-8-hydroxy butyric acid (GABOB), an antiepileptic and hypotensive drug .
Molecular Structure Analysis
The molecular structure of 1,2,4-Butanetriol can be viewed using Java or Javascript .
Chemical Reactions Analysis
1,2,4-Butanetriol is an important non-natural chemical with a variety of industrial applications. Identifying the bottlenecks for BT biosynthesis is expected to contribute to improving the efficiency of this process .
Physical And Chemical Properties Analysis
1,2,4-Butanetriol is a clear or slightly yellow, odorless, hygroscopic, flammable, viscous liquid. It is an alcohol with three hydrophilic alcoholic hydroxyl groups. It is similar to glycerol and erythritol. It is chiral, with two possible enantiomers .
Scientific Research Applications
Military Industry Applications
Due to its properties, 1,2,4-Butanetriol is utilized in the military industry for the synthesis of energetic materials such as 1,2,4-butanetriol trinitrate .
Production of Solvents
This chemical is also used in the production of solvents that have applications across various fields .
Cholesterol-Lowering Drugs
It has been reported that 1,2,4-Butanetriol can be used to synthesize cholesterol-lowering drugs, expanding its importance in the pharmaceutical industry .
Polyurethane Manufacturing
1,2,4-Butanetriol is a raw material for making polyurethane foams with better elastic properties. This application is significant in materials science and engineering .
Biosynthesis Research
There is ongoing research into the biosynthesis of 1,2,4-Butanetriol from different sugars like xylose and D-arabinose. This research has implications for sustainable chemical production and biotechnology .
Mechanism of Action
Target of Action
1,2,4-Butanetriol (BT) is an important non-natural chemical with a variety of industrial applications . It is used in the synthesis of 1,2,4-butanetriol trinitrate (BTTN), an important military propellant . It can also be used as the raw material to synthesize polymeric materials and medical precursors for drug delivery . The primary targets of BT are therefore the biochemical pathways that lead to these end products.
Mode of Action
The mode of action of BT involves its interaction with various enzymes in the biochemical pathways that lead to its end products. For instance, in the biosynthesis of BT from d-xylose, BT is produced from d-xylose or l-arabinose catalyzed sequentially by Pseudomonas fragi and Escherichia coli . This process was further simplified using metabolic engineered E. coli strains .
Biochemical Pathways
The biosynthesis of BT involves a four-step synthetic pathway and the disruption of competing pathways for xylose in Escherichia coli BW25113 . The entire BT synthetic pathway is introduced while blocking the competing pathways . The biosynthetic pathway establishment for BT from xylose, metabolic engineering strategies employed for improving BT production from xylose, and other substrates for BT production have been studied .
Pharmacokinetics
Information on the ADME (Absorption, Distribution, Metabolism, and Excretion) properties of BT is currently limited. It is known that bt is a clear or slightly yellow, odorless, hygroscopic, flammable, viscous liquid . It is an alcohol with three hydrophilic alcoholic hydroxyl groups, which suggests that it may be readily absorbed and distributed in the body .
Result of Action
The molecular and cellular effects of BT’s action are primarily related to its role as a precursor in the synthesis of various end products. For instance, it is used in the synthesis of 1,2,4-butanetriol trinitrate (BTTN), an important military propellant . It can also be used as the raw material to synthesize polymeric materials and medical precursors for drug delivery .
Action Environment
The action, efficacy, and stability of BT can be influenced by various environmental factors. For instance, the production conditions for BT were optimized by exploring the effects of temperature, pH, and cell density on BT titer . Furthermore, the biotechnological synthesis of BT has been considered a viable alternative to traditional chemical synthesis methods due to its advantages of mild reaction conditions and green sustainability .
Safety and Hazards
Future Directions
properties
IUPAC Name |
butane-1,2,4-triol | |
---|---|---|
Source | PubChem | |
URL | https://pubchem.ncbi.nlm.nih.gov | |
Description | Data deposited in or computed by PubChem | |
InChI |
InChI=1S/C4H10O3/c5-2-1-4(7)3-6/h4-7H,1-3H2 | |
Source | PubChem | |
URL | https://pubchem.ncbi.nlm.nih.gov | |
Description | Data deposited in or computed by PubChem | |
InChI Key |
ARXKVVRQIIOZGF-UHFFFAOYSA-N | |
Source | PubChem | |
URL | https://pubchem.ncbi.nlm.nih.gov | |
Description | Data deposited in or computed by PubChem | |
Canonical SMILES |
C(CO)C(CO)O | |
Source | PubChem | |
URL | https://pubchem.ncbi.nlm.nih.gov | |
Description | Data deposited in or computed by PubChem | |
Molecular Formula |
C4H10O3 | |
Source | PubChem | |
URL | https://pubchem.ncbi.nlm.nih.gov | |
Description | Data deposited in or computed by PubChem | |
DSSTOX Substance ID |
DTXSID8044416 | |
Record name | 1,2,4-Butanetriol | |
Source | EPA DSSTox | |
URL | https://comptox.epa.gov/dashboard/DTXSID8044416 | |
Description | DSSTox provides a high quality public chemistry resource for supporting improved predictive toxicology. | |
Molecular Weight |
106.12 g/mol | |
Source | PubChem | |
URL | https://pubchem.ncbi.nlm.nih.gov | |
Description | Data deposited in or computed by PubChem | |
Physical Description |
Colorless to yellow liquid, miscible in water; [MSDSonline] | |
Record name | 1,2,4-Butanetriol | |
Source | Haz-Map, Information on Hazardous Chemicals and Occupational Diseases | |
URL | https://haz-map.com/Agents/7888 | |
Description | Haz-Map® is an occupational health database designed for health and safety professionals and for consumers seeking information about the adverse effects of workplace exposures to chemical and biological agents. | |
Explanation | Copyright (c) 2022 Haz-Map(R). All rights reserved. Unless otherwise indicated, all materials from Haz-Map are copyrighted by Haz-Map(R). No part of these materials, either text or image may be used for any purpose other than for personal use. Therefore, reproduction, modification, storage in a retrieval system or retransmission, in any form or by any means, electronic, mechanical or otherwise, for reasons other than personal use, is strictly prohibited without prior written permission. | |
Product Name |
1,2,4-Butanetriol | |
CAS RN |
3068-00-6 | |
Record name | 1,2,4-Butanetriol | |
Source | CAS Common Chemistry | |
URL | https://commonchemistry.cas.org/detail?cas_rn=3068-00-6 | |
Description | CAS Common Chemistry is an open community resource for accessing chemical information. Nearly 500,000 chemical substances from CAS REGISTRY cover areas of community interest, including common and frequently regulated chemicals, and those relevant to high school and undergraduate chemistry classes. This chemical information, curated by our expert scientists, is provided in alignment with our mission as a division of the American Chemical Society. | |
Explanation | The data from CAS Common Chemistry is provided under a CC-BY-NC 4.0 license, unless otherwise stated. | |
Record name | 1,3,4-Butanetriol | |
Source | ChemIDplus | |
URL | https://pubchem.ncbi.nlm.nih.gov/substance/?source=chemidplus&sourceid=0003068006 | |
Description | ChemIDplus is a free, web search system that provides access to the structure and nomenclature authority files used for the identification of chemical substances cited in National Library of Medicine (NLM) databases, including the TOXNET system. | |
Record name | 2-Deoxyerythritol | |
Source | DTP/NCI | |
URL | https://dtp.cancer.gov/dtpstandard/servlet/dwindex?searchtype=NSC&outputformat=html&searchlist=60197 | |
Description | The NCI Development Therapeutics Program (DTP) provides services and resources to the academic and private-sector research communities worldwide to facilitate the discovery and development of new cancer therapeutic agents. | |
Explanation | Unless otherwise indicated, all text within NCI products is free of copyright and may be reused without our permission. Credit the National Cancer Institute as the source. | |
Record name | 1,2,4-Butanetriol | |
Source | EPA Chemicals under the TSCA | |
URL | https://www.epa.gov/chemicals-under-tsca | |
Description | EPA Chemicals under the Toxic Substances Control Act (TSCA) collection contains information on chemicals and their regulations under TSCA, including non-confidential content from the TSCA Chemical Substance Inventory and Chemical Data Reporting. | |
Record name | 1,2,4-Butanetriol | |
Source | EPA DSSTox | |
URL | https://comptox.epa.gov/dashboard/DTXSID8044416 | |
Description | DSSTox provides a high quality public chemistry resource for supporting improved predictive toxicology. | |
Record name | Butane-1,2,4-triol | |
Source | European Chemicals Agency (ECHA) | |
URL | https://echa.europa.eu/substance-information/-/substanceinfo/100.019.385 | |
Description | The European Chemicals Agency (ECHA) is an agency of the European Union which is the driving force among regulatory authorities in implementing the EU's groundbreaking chemicals legislation for the benefit of human health and the environment as well as for innovation and competitiveness. | |
Explanation | Use of the information, documents and data from the ECHA website is subject to the terms and conditions of this Legal Notice, and subject to other binding limitations provided for under applicable law, the information, documents and data made available on the ECHA website may be reproduced, distributed and/or used, totally or in part, for non-commercial purposes provided that ECHA is acknowledged as the source: "Source: European Chemicals Agency, http://echa.europa.eu/". Such acknowledgement must be included in each copy of the material. ECHA permits and encourages organisations and individuals to create links to the ECHA website under the following cumulative conditions: Links can only be made to webpages that provide a link to the Legal Notice page. | |
Record name | 1,2,4-BUTANETRIOL | |
Source | FDA Global Substance Registration System (GSRS) | |
URL | https://gsrs.ncats.nih.gov/ginas/app/beta/substances/NK798C370H | |
Description | The FDA Global Substance Registration System (GSRS) enables the efficient and accurate exchange of information on what substances are in regulated products. Instead of relying on names, which vary across regulatory domains, countries, and regions, the GSRS knowledge base makes it possible for substances to be defined by standardized, scientific descriptions. | |
Explanation | Unless otherwise noted, the contents of the FDA website (www.fda.gov), both text and graphics, are not copyrighted. They are in the public domain and may be republished, reprinted and otherwise used freely by anyone without the need to obtain permission from FDA. Credit to the U.S. Food and Drug Administration as the source is appreciated but not required. | |
Synthesis routes and methods I
Procedure details
Synthesis routes and methods II
Procedure details
Retrosynthesis Analysis
AI-Powered Synthesis Planning: Our tool employs the Template_relevance Pistachio, Template_relevance Bkms_metabolic, Template_relevance Pistachio_ringbreaker, Template_relevance Reaxys, Template_relevance Reaxys_biocatalysis model, leveraging a vast database of chemical reactions to predict feasible synthetic routes.
One-Step Synthesis Focus: Specifically designed for one-step synthesis, it provides concise and direct routes for your target compounds, streamlining the synthesis process.
Accurate Predictions: Utilizing the extensive PISTACHIO, BKMS_METABOLIC, PISTACHIO_RINGBREAKER, REAXYS, REAXYS_BIOCATALYSIS database, our tool offers high-accuracy predictions, reflecting the latest in chemical research and data.
Strategy Settings
Precursor scoring | Relevance Heuristic |
---|---|
Min. plausibility | 0.01 |
Model | Template_relevance |
Template Set | Pistachio/Bkms_metabolic/Pistachio_ringbreaker/Reaxys/Reaxys_biocatalysis |
Top-N result to add to graph | 6 |
Feasible Synthetic Routes
Disclaimer and Information on In-Vitro Research Products
Please be aware that all articles and product information presented on BenchChem are intended solely for informational purposes. The products available for purchase on BenchChem are specifically designed for in-vitro studies, which are conducted outside of living organisms. In-vitro studies, derived from the Latin term "in glass," involve experiments performed in controlled laboratory settings using cells or tissues. It is important to note that these products are not categorized as medicines or drugs, and they have not received approval from the FDA for the prevention, treatment, or cure of any medical condition, ailment, or disease. We must emphasize that any form of bodily introduction of these products into humans or animals is strictly prohibited by law. It is essential to adhere to these guidelines to ensure compliance with legal and ethical standards in research and experimentation.