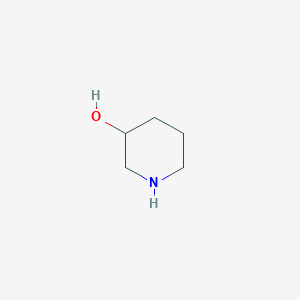
3-Hydroxypiperidine
Overview
Description
This compound is a derivative of piperidine and is known for its significant role in the synthesis of various pharmaceuticals and bioactive molecules .
Synthetic Routes and Reaction Conditions:
Reduction of 3-Hydroxypyridine: One common method involves the reduction of 3-hydroxypyridine using hydrogenation catalysts such as rhodium on carbon (Rh/C) to yield 3-hydroxypiperidine.
Industrial Production Methods:
Chemical Synthesis: Industrial production often involves the chemical synthesis route using hydrogenation of 3-hydroxypyridine.
Biotechnological Methods: The use of thermostable aldo-keto reductase enzymes in bioreactors is another industrial method, offering high enantioselectivity and efficiency.
Types of Reactions:
Common Reagents and Conditions:
Oxidizing Agents: Iodosylbenzene for oxidation reactions.
Substitution Reagents: 4-Bromoquinoline for substitution reactions.
Major Products:
2-Pyrrolidinone: Formed via oxidation.
1-[2,8-Bis(trifluoromethyl)quinolin-4-yl]piperidin-3-ol: Formed via substitution.
Mechanism of Action
Target of Action
3-Hydroxypiperidine, also known as Piperidin-3-ol, is a key pharmaceutical intermediate . It is used in the synthesis of Imbruvica, a drug used in lymphoma therapy . Imbruvica targets Bruton’s tyrosine kinase (BTK), a crucial player in B-cell development . Therefore, the primary target of this compound, through its role in Imbruvica, is BTK .
Mode of Action
Its derivative, imbruvica, works by irreversibly binding to btk, inhibiting its activity . This inhibition disrupts B-cell receptor signaling, leading to decreased survival and proliferation of malignant B-cells .
Biochemical Pathways
This compound is involved in the synthesis of various compounds . For instance, it can be used to synthesize 2-pyrrolidinone via oxidation with iodosylbenzene . It can also react with 4-bromoquinoline to produce 1-[2,8-bis(trifluoromethyl)quinolin-4-yl]piperidin-3-ol . These reactions indicate that this compound can participate in various biochemical pathways, contributing to the synthesis of a range of bioactive molecules.
Pharmacokinetics
Given its solubility in water , it can be inferred that it may have good bioavailability
Result of Action
Its role as a precursor in the synthesis of imbruvica suggests that it contributes to the therapeutic effects of this drug . Imbruvica has been shown to effectively inhibit the growth and survival of malignant B-cells, leading to its use in lymphoma therapy .
Action Environment
It is known that the compound is stable under normal storage conditions (2-8°c)
Biochemical Analysis
Biochemical Properties
3-Hydroxypiperidine plays a crucial role in various biochemical reactions. It is known to interact with several enzymes, proteins, and other biomolecules. One notable interaction is with carbonyl reductase enzymes, which catalyze the reduction of carbonyl compounds to their corresponding alcohols. For instance, an ®-specific carbonyl reductase from Candida parapsilosis has been identified to catalyze the asymmetric reduction of this compound, producing chiral intermediates used in pharmaceutical synthesis .
Additionally, this compound is involved in the synthesis of piperidine nucleoside analogs and substituted pyridines, highlighting its importance in medicinal chemistry . The nature of these interactions often involves hydrogen bonding and hydrophobic interactions, which stabilize the compound within the active sites of enzymes.
Cellular Effects
This compound influences various cellular processes and functions. It has been observed to affect cell signaling pathways, gene expression, and cellular metabolism. For example, in the synthesis of (S)-N-Boc-3-hydroxypiperidine, the compound acts as a chiral intermediate, influencing the activity of Bruton’s tyrosine kinase (BTK), a key enzyme in cell signaling pathways related to lymphoma therapy .
Moreover, this compound’s impact on cellular metabolism is evident in its role in the biocatalytic reduction processes, where it serves as a substrate for enzymes like ketoreductase and glucose dehydrogenase. These interactions can lead to changes in metabolic flux and the levels of various metabolites within the cell .
Molecular Mechanism
The molecular mechanism of this compound involves its binding interactions with specific biomolecules. For instance, the compound binds to the active site of aldo-keto reductase (AKR) enzymes, facilitating the reduction of N-Boc-3-piperidone to (S)-N-Boc-3-hydroxypiperidine . This process is characterized by a classic ordered two-step catalytic mechanism, where the enzyme first binds to the substrate, followed by the reduction reaction facilitated by cofactor recycling through glucose dehydrogenase.
Molecular simulations have demonstrated the structural rationale for the enantioselectivity of AKR-43 toward N-Boc-3-piperidone, supporting the high specificity and efficiency of this biocatalytic process .
Temporal Effects in Laboratory Settings
In laboratory settings, the effects of this compound can change over time due to factors such as stability and degradation. Studies have shown that the compound remains stable under optimal conditions, such as a temperature of 30°C and pH 7.5, for up to 16 hours . Prolonged exposure to suboptimal conditions can lead to degradation, affecting its efficacy in biochemical reactions.
Long-term effects on cellular function have been observed in in vitro studies, where the continuous presence of this compound can lead to sustained changes in enzyme activity and metabolic pathways .
Dosage Effects in Animal Models
The effects of this compound vary with different dosages in animal models. At low doses, the compound has been shown to have minimal adverse effects, while higher doses can lead to toxicity and adverse reactions. For instance, studies on the dosage effects of similar compounds have demonstrated threshold effects, where a certain dosage level is required to achieve the desired therapeutic effect without causing toxicity .
In animal models, high doses of this compound can lead to hepatotoxicity and neurotoxic effects, emphasizing the importance of careful dosage optimization in therapeutic applications .
Metabolic Pathways
This compound is involved in several metabolic pathways, including the degradation of nicotinate in microbial eukaryotes. In Aspergillus nidulans, the compound is converted through a series of enzymatic steps, resulting in the formation of metabolites such as this compound-2,6-dione and 5,6-dihydroxypiperidine-2-one . These pathways highlight the compound’s role in nitrogen and carbon metabolism.
The enzymes involved in these pathways include hydroxylases and dehydrogenases, which facilitate the conversion of this compound into various intermediates and end products .
Transport and Distribution
Within cells and tissues, this compound is transported and distributed through interactions with specific transporters and binding proteins. The compound’s solubility in water allows it to be readily absorbed and distributed in aqueous environments . Additionally, its interactions with binding proteins can influence its localization and accumulation within specific cellular compartments .
Subcellular Localization
The subcellular localization of this compound is influenced by targeting signals and post-translational modifications. The compound is often directed to specific compartments or organelles, such as the cytoplasm or mitochondria, where it exerts its biochemical effects . These targeting signals ensure that this compound reaches the appropriate location within the cell to participate in metabolic and signaling pathways.
Scientific Research Applications
3-Hydroxypiperidine is widely used in various fields of scientific research:
Chemistry: It serves as a building block for the synthesis of complex organic molecules and pharmaceuticals.
Biology: It is used in the study of enzyme mechanisms and as a substrate in enzymatic reactions.
Medicine: It is a key intermediate in the synthesis of drugs like ibrutinib, which is used to treat lymphoma.
Comparison with Similar Compounds
4-Hydroxypiperidine: Another hydroxylated piperidine derivative with similar chemical properties but different biological activities.
1-Boc-3-hydroxypiperidine: A protected form of 3-hydroxypiperidine used in various synthetic applications.
Uniqueness:
Properties
IUPAC Name |
piperidin-3-ol | |
---|---|---|
Source | PubChem | |
URL | https://pubchem.ncbi.nlm.nih.gov | |
Description | Data deposited in or computed by PubChem | |
InChI |
InChI=1S/C5H11NO/c7-5-2-1-3-6-4-5/h5-7H,1-4H2 | |
Source | PubChem | |
URL | https://pubchem.ncbi.nlm.nih.gov | |
Description | Data deposited in or computed by PubChem | |
InChI Key |
BIWOSRSKDCZIFM-UHFFFAOYSA-N | |
Source | PubChem | |
URL | https://pubchem.ncbi.nlm.nih.gov | |
Description | Data deposited in or computed by PubChem | |
Canonical SMILES |
C1CC(CNC1)O | |
Source | PubChem | |
URL | https://pubchem.ncbi.nlm.nih.gov | |
Description | Data deposited in or computed by PubChem | |
Molecular Formula |
C5H11NO | |
Source | PubChem | |
URL | https://pubchem.ncbi.nlm.nih.gov | |
Description | Data deposited in or computed by PubChem | |
DSSTOX Substance ID |
DTXSID70870212 | |
Record name | 3-Piperidinol | |
Source | EPA DSSTox | |
URL | https://comptox.epa.gov/dashboard/DTXSID70870212 | |
Description | DSSTox provides a high quality public chemistry resource for supporting improved predictive toxicology. | |
Molecular Weight |
101.15 g/mol | |
Source | PubChem | |
URL | https://pubchem.ncbi.nlm.nih.gov | |
Description | Data deposited in or computed by PubChem | |
CAS No. |
6859-99-0 | |
Record name | 3-Hydroxypiperidine | |
Source | CAS Common Chemistry | |
URL | https://commonchemistry.cas.org/detail?cas_rn=6859-99-0 | |
Description | CAS Common Chemistry is an open community resource for accessing chemical information. Nearly 500,000 chemical substances from CAS REGISTRY cover areas of community interest, including common and frequently regulated chemicals, and those relevant to high school and undergraduate chemistry classes. This chemical information, curated by our expert scientists, is provided in alignment with our mission as a division of the American Chemical Society. | |
Explanation | The data from CAS Common Chemistry is provided under a CC-BY-NC 4.0 license, unless otherwise stated. | |
Record name | Piperidin-3-ol | |
Source | ChemIDplus | |
URL | https://pubchem.ncbi.nlm.nih.gov/substance/?source=chemidplus&sourceid=0006859990 | |
Description | ChemIDplus is a free, web search system that provides access to the structure and nomenclature authority files used for the identification of chemical substances cited in National Library of Medicine (NLM) databases, including the TOXNET system. | |
Record name | 3-Hydroxypiperidine | |
Source | DTP/NCI | |
URL | https://dtp.cancer.gov/dtpstandard/servlet/dwindex?searchtype=NSC&outputformat=html&searchlist=62082 | |
Description | The NCI Development Therapeutics Program (DTP) provides services and resources to the academic and private-sector research communities worldwide to facilitate the discovery and development of new cancer therapeutic agents. | |
Explanation | Unless otherwise indicated, all text within NCI products is free of copyright and may be reused without our permission. Credit the National Cancer Institute as the source. | |
Record name | 3-Piperidinol | |
Source | EPA DSSTox | |
URL | https://comptox.epa.gov/dashboard/DTXSID70870212 | |
Description | DSSTox provides a high quality public chemistry resource for supporting improved predictive toxicology. | |
Record name | Piperidin-3-ol | |
Source | European Chemicals Agency (ECHA) | |
URL | https://echa.europa.eu/substance-information/-/substanceinfo/100.027.234 | |
Description | The European Chemicals Agency (ECHA) is an agency of the European Union which is the driving force among regulatory authorities in implementing the EU's groundbreaking chemicals legislation for the benefit of human health and the environment as well as for innovation and competitiveness. | |
Explanation | Use of the information, documents and data from the ECHA website is subject to the terms and conditions of this Legal Notice, and subject to other binding limitations provided for under applicable law, the information, documents and data made available on the ECHA website may be reproduced, distributed and/or used, totally or in part, for non-commercial purposes provided that ECHA is acknowledged as the source: "Source: European Chemicals Agency, http://echa.europa.eu/". Such acknowledgement must be included in each copy of the material. ECHA permits and encourages organisations and individuals to create links to the ECHA website under the following cumulative conditions: Links can only be made to webpages that provide a link to the Legal Notice page. | |
Retrosynthesis Analysis
AI-Powered Synthesis Planning: Our tool employs the Template_relevance Pistachio, Template_relevance Bkms_metabolic, Template_relevance Pistachio_ringbreaker, Template_relevance Reaxys, Template_relevance Reaxys_biocatalysis model, leveraging a vast database of chemical reactions to predict feasible synthetic routes.
One-Step Synthesis Focus: Specifically designed for one-step synthesis, it provides concise and direct routes for your target compounds, streamlining the synthesis process.
Accurate Predictions: Utilizing the extensive PISTACHIO, BKMS_METABOLIC, PISTACHIO_RINGBREAKER, REAXYS, REAXYS_BIOCATALYSIS database, our tool offers high-accuracy predictions, reflecting the latest in chemical research and data.
Strategy Settings
Precursor scoring | Relevance Heuristic |
---|---|
Min. plausibility | 0.01 |
Model | Template_relevance |
Template Set | Pistachio/Bkms_metabolic/Pistachio_ringbreaker/Reaxys/Reaxys_biocatalysis |
Top-N result to add to graph | 6 |
Feasible Synthetic Routes
Q1: What is the molecular formula and weight of 3-hydroxypiperidine?
A1: this compound has the molecular formula C5H11NO and a molecular weight of 101.15 g/mol.
Q2: Is there any spectroscopic data available for this compound?
A2: Yes, studies have used NMR spectroscopy to determine the conformational equilibrium of 1-alkyl-3-hydroxypiperidines in different solvents. This technique helps analyze the preferred conformations of the molecule in various environments.
Q3: What are some synthetic approaches to access this compound derivatives?
A3: Several strategies exist to synthesize this compound derivatives:
- Chiral Resolution: Racemic mixtures of this compound can be resolved using resolving agents like D-pyroglutamic acid or derivatives of D-tartaric acid.
- Biocatalysis: Baker's yeast, ketoreductase enzymes, and microbial cells have been explored for the enantioselective synthesis of (S)-1-Boc-3-hydroxypiperidine. These methods offer environmentally friendly alternatives to traditional chemical synthesis.
- Ring Expansion: Optically active 3-hydroxypiperidines can be obtained from pyrrolidinemethanol derivatives via a ring expansion reaction using trifluoroacetic anhydride.
- Reductive Cleavage: N-Carboxymethyl this compound can undergo substitution at the C6 position via reductive cleavage of N,S-acetals, providing access to 2-substituted-5-hydroxypiperidines.
- Diastereoselective Synthesis: Approaches involving radical cyclization reactions have been developed for the diastereoselective synthesis of 2,5-disubstituted 3-hydroxypyrrolidine and 2,6-disubstituted this compound derivatives.
Q4: Are there chemoenzymatic approaches to synthesize enantiopure febrifugine, a natural product containing the this compound moiety?
A4: Yes, researchers have developed two complementary chemoenzymatic strategies to synthesize both enantiomers of febrifugine. These methods utilize previously established chemoenzymatic approaches to construct the this compound core and incorporate the quinazolone-containing side chain through an N-acyliminium ion-mediated coupling reaction.
Q5: Can you elaborate on the biocatalytic synthesis of (S)-N-Boc-3-hydroxypiperidine?
A5: (S)-N-Boc-3-hydroxypiperidine, a crucial chiral intermediate for synthesizing pharmaceuticals like ibrutinib, can be produced through biocatalytic reduction. This process utilizes ketoreductase enzymes, often in conjunction with glucose dehydrogenase, to convert N-Boc-piperidin-3-one to the desired (S)-enantiomer. Optimization of this process using recombinant enzymes and suitable reaction conditions allows for high substrate concentrations, excellent enantiomeric excess (>99%), and minimal enzyme loading, demonstrating its commercial potential.
Q6: What is the significance of immobilized whole-cell compositions in the synthesis of (S)-N-t-butyloxycarbonyl-3-hydroxypiperidine?
A6: Immobilized whole-cell compositions, containing the necessary biocatalysts, offer several advantages for synthesizing (S)-N-t-butyloxycarbonyl-3-hydroxypiperidine. These benefits include reduced cost, simplified operation, and the crucial ability to recycle the catalysts, making this method highly attractive for industrial applications.
Q7: What are the key applications of this compound?
A7: this compound serves as a versatile building block in organic synthesis, particularly in medicinal chemistry. It represents a key structural motif in many bioactive compounds and natural products, including pharmaceuticals and agrochemicals.
Q8: How does the structure of this compound relate to its use in drug discovery?
A8: The this compound scaffold exhibits a privileged structure in medicinal chemistry. Its presence in various natural products with diverse biological activities makes it an attractive target for the development of new drugs. The presence of the hydroxyl group at the 3-position allows for further derivatization and fine-tuning of the molecule's pharmacological properties.
Q9: Can you provide specific examples of this compound derivatives with biological activity?
A9: Certainly!
- (S)-N-Boc-3-hydroxypiperidine: This compound is a vital intermediate in synthesizing ibrutinib, a medication used to treat certain types of cancer.
- Derivatives as NMDA Receptor Blockers: Specific this compound derivatives have shown potential as prodrugs for treating diseases associated with NMDA receptor dysfunction.
- Piperlongumine Derivatives: Synthetic piperlongumine derivatives, incorporating the this compound moiety, exhibited inhibitory activity against aflatoxin B1 production, suggesting potential applications in controlling mycotoxins in agricultural products.
Q10: Can you provide details on the metabolism of N-ethyl-3-piperidyl benzilate, a compound containing the this compound moiety?
A10: Studies on the metabolic fate of N-ethyl-3-piperidyl benzilate (I) in rats revealed that it undergoes hydrolysis to form 3-piperidyl benzilate (II) and N-ethyl-3-hydroxypiperidine (III). Further metabolic transformations and excretion pathways are still under investigation.
Disclaimer and Information on In-Vitro Research Products
Please be aware that all articles and product information presented on BenchChem are intended solely for informational purposes. The products available for purchase on BenchChem are specifically designed for in-vitro studies, which are conducted outside of living organisms. In-vitro studies, derived from the Latin term "in glass," involve experiments performed in controlled laboratory settings using cells or tissues. It is important to note that these products are not categorized as medicines or drugs, and they have not received approval from the FDA for the prevention, treatment, or cure of any medical condition, ailment, or disease. We must emphasize that any form of bodily introduction of these products into humans or animals is strictly prohibited by law. It is essential to adhere to these guidelines to ensure compliance with legal and ethical standards in research and experimentation.