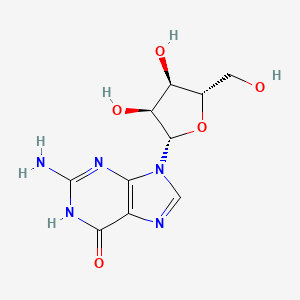
L-Guanosine
Overview
Description
L-Guanosine is a purine nucleoside composed of guanine attached to a ribose (ribofuranose) ring via a β-N9-glycosidic bond . It plays a crucial role in various biochemical processes, including nucleic acid synthesis, signal transduction, and cellular metabolism. This compound is known for its neuroprotective properties and is released in the brain under both physiological and pathological conditions .
Mechanism of Action
Target of Action
L-Guanosine, a purine nucleoside, primarily targets the central nervous system (CNS). It acts as a neuromodulator mediating several cellular processes, including cell growth, differentiation, and survival . It also interacts with adenosine receptors, which are composed of four different G-protein-coupled receptors (GPCRs) - A1R, A2AR, A2BR, and A3R .
Mode of Action
This compound interacts with its targets, leading to various changes. The intracellular signaling pathways related to this compound effects were the first targets to be identified. It was shown that this compound’s effect on cell proliferation is dependent on cyclic AMP (cAMP) level increase . It also interacts with well-known membrane proteins in glutamatergic and adenosinergic systems .
Biochemical Pathways
This compound affects several biochemical pathways. It modulates glutamate uptake, decreases the production of reactive oxygen species (ROS), and improves mitochondrial function . It also presents anti-inflammatory properties . This compound can be phosphorylated to become GMP (guanosine monophosphate), cGMP (cyclic guanosine monophosphate), GDP (guanosine diphosphate), and GTP (guanosine triphosphate), which are factors in signal transduction pathways .
Pharmacokinetics
It is known that this compound is released in the brain under physiological conditions and even more during pathological events .
Result of Action
This compound exerts protective effects in several models of neurotoxicity or neurological disorders (both in vitro and in vivo). It presents anxiolytic, antidepressant-like, antinociceptive, and anticonvulsant effects in rodents . This compound treatment exerts neuroprotection on Alzheimer’s and Parkinson’s disease in vivo models, ameliorating behavior, cognitive, and motor function . In vitro studies showed that this compound increases the number of neurons in culture and promotes neural stem cell proliferation and neuronal differentiation .
Biochemical Analysis
Biochemical Properties
L-Guanosine participates in several biochemical reactions, primarily through its conversion to guanosine monophosphate (GMP) and subsequently to GTP. It interacts with enzymes such as guanosine kinase, which phosphorylates this compound to GMP. Additionally, this compound is involved in the purine salvage pathway, where it is recycled to form nucleotides. The interactions of this compound with these enzymes are critical for maintaining nucleotide pools within the cell .
Cellular Effects
This compound influences various cellular processes, including cell signaling, gene expression, and metabolism. It acts as a signaling molecule in the purinergic signaling pathway, where it binds to specific receptors on the cell surface, triggering downstream signaling cascades. These pathways can affect gene expression and cellular metabolism, leading to changes in cell function. For example, this compound has been shown to reduce neuroinflammation and oxidative stress in neuronal cells .
Molecular Mechanism
At the molecular level, this compound exerts its effects through binding interactions with specific biomolecules. It can activate or inhibit enzymes involved in nucleotide metabolism, such as guanosine kinase and GMP synthetase. Additionally, this compound can modulate gene expression by influencing transcription factors and other regulatory proteins. These interactions are essential for the compound’s role in cellular processes and its therapeutic potential .
Temporal Effects in Laboratory Settings
In laboratory settings, the effects of this compound can change over time due to its stability and degradation. Studies have shown that this compound is relatively stable under physiological conditions, but it can degrade over time, leading to a decrease in its effectiveness. Long-term exposure to this compound has been observed to have sustained effects on cellular function, including prolonged activation of signaling pathways and changes in gene expression .
Dosage Effects in Animal Models
The effects of this compound vary with different dosages in animal models. At low doses, this compound has been shown to have neuroprotective effects, reducing neuroinflammation and oxidative stress. At high doses, it can have toxic effects, leading to cell death and tissue damage. These threshold effects highlight the importance of careful dosage optimization in therapeutic applications .
Metabolic Pathways
This compound is involved in several metabolic pathways, including the purine salvage pathway and the de novo synthesis of nucleotides. It interacts with enzymes such as guanosine kinase, GMP synthetase, and inosine monophosphate dehydrogenase. These interactions are crucial for maintaining the balance of nucleotide pools and ensuring proper cellular function .
Transport and Distribution
Within cells, this compound is transported and distributed through specific transporters and binding proteins. These transporters facilitate the uptake of this compound into cells and its distribution to various cellular compartments. The localization and accumulation of this compound can affect its activity and function, influencing cellular processes such as signal transduction and metabolism .
Subcellular Localization
This compound is localized in various subcellular compartments, including the cytoplasm and nucleus. Its activity and function can be influenced by post-translational modifications and targeting signals that direct it to specific organelles. For example, this compound can be phosphorylated to GMP in the cytoplasm and then transported to the nucleus, where it participates in nucleic acid synthesis .
Preparation Methods
Synthetic Routes and Reaction Conditions: L-Guanosine can be synthesized through various methods, including chemical synthesis and microbial fermentation. One common synthetic route involves the reaction of guanine with ribose in the presence of an acid catalyst to form the β-N9-glycosidic bond . Another method involves the use of phosphoribosyltransferases to catalyze the formation of guanosine from guanine and ribose-5-phosphate .
Industrial Production Methods: Industrial production of this compound often relies on microbial fermentation using genetically engineered strains of Escherichia coli. These strains are optimized to overexpress the purine synthesis pathway and other relevant enzymes to enhance guanosine production . The fermentation process is typically carried out in fed-batch reactors to achieve high yields.
Chemical Reactions Analysis
Types of Reactions: L-Guanosine undergoes various chemical reactions, including oxidation, reduction, and substitution. For example, it can be phosphorylated to form guanosine monophosphate, cyclic guanosine monophosphate, guanosine diphosphate, and guanosine triphosphate .
Common Reagents and Conditions:
Oxidation: this compound can be oxidized using reagents such as potassium permanganate or hydrogen peroxide under acidic conditions.
Reduction: Reduction reactions can be carried out using reducing agents like sodium borohydride.
Substitution: Substitution reactions often involve nucleophilic substitution using reagents like alkyl halides or acyl chlorides.
Major Products: The major products formed from these reactions include various phosphorylated derivatives of guanosine, which play essential roles in cellular signaling and metabolism .
Scientific Research Applications
L-Guanosine has extensive applications in scientific research, particularly in the fields of chemistry, biology, medicine, and industry . Some of its notable applications include:
Chemistry: Used as a precursor for the synthesis of other nucleoside analogs and derivatives.
Biology: Plays a role in nucleic acid synthesis and cellular signaling pathways.
Industry: Used in the production of food additives and pharmaceutical products.
Comparison with Similar Compounds
L-Guanosine is unique among purine nucleosides due to its specific neuroprotective properties and interactions with adenosine receptors . Similar compounds include:
Adenosine: Another purine nucleoside that acts as a neuromodulator and has neuroprotective effects.
Inosine: A purine nucleoside with anti-inflammatory and neuroprotective properties.
Cytidine: A pyrimidine nucleoside involved in nucleic acid synthesis and cellular metabolism.
This compound stands out due to its specific interactions with adenosine receptors and its potential therapeutic applications in neurodegenerative diseases .
Properties
IUPAC Name |
2-amino-9-[(2S,3S,4R,5S)-3,4-dihydroxy-5-(hydroxymethyl)oxolan-2-yl]-1H-purin-6-one | |
---|---|---|
Source | PubChem | |
URL | https://pubchem.ncbi.nlm.nih.gov | |
Description | Data deposited in or computed by PubChem | |
InChI |
InChI=1S/C10H13N5O5/c11-10-13-7-4(8(19)14-10)12-2-15(7)9-6(18)5(17)3(1-16)20-9/h2-3,5-6,9,16-18H,1H2,(H3,11,13,14,19)/t3-,5-,6-,9-/m0/s1 | |
Source | PubChem | |
URL | https://pubchem.ncbi.nlm.nih.gov | |
Description | Data deposited in or computed by PubChem | |
InChI Key |
NYHBQMYGNKIUIF-GIMIYPNGSA-N | |
Source | PubChem | |
URL | https://pubchem.ncbi.nlm.nih.gov | |
Description | Data deposited in or computed by PubChem | |
Canonical SMILES |
C1=NC2=C(N1C3C(C(C(O3)CO)O)O)N=C(NC2=O)N | |
Source | PubChem | |
URL | https://pubchem.ncbi.nlm.nih.gov | |
Description | Data deposited in or computed by PubChem | |
Isomeric SMILES |
C1=NC2=C(N1[C@@H]3[C@H]([C@H]([C@@H](O3)CO)O)O)N=C(NC2=O)N | |
Source | PubChem | |
URL | https://pubchem.ncbi.nlm.nih.gov | |
Description | Data deposited in or computed by PubChem | |
Molecular Formula |
C10H13N5O5 | |
Source | PubChem | |
URL | https://pubchem.ncbi.nlm.nih.gov | |
Description | Data deposited in or computed by PubChem | |
Molecular Weight |
283.24 g/mol | |
Source | PubChem | |
URL | https://pubchem.ncbi.nlm.nih.gov | |
Description | Data deposited in or computed by PubChem | |
Retrosynthesis Analysis
AI-Powered Synthesis Planning: Our tool employs the Template_relevance Pistachio, Template_relevance Bkms_metabolic, Template_relevance Pistachio_ringbreaker, Template_relevance Reaxys, Template_relevance Reaxys_biocatalysis model, leveraging a vast database of chemical reactions to predict feasible synthetic routes.
One-Step Synthesis Focus: Specifically designed for one-step synthesis, it provides concise and direct routes for your target compounds, streamlining the synthesis process.
Accurate Predictions: Utilizing the extensive PISTACHIO, BKMS_METABOLIC, PISTACHIO_RINGBREAKER, REAXYS, REAXYS_BIOCATALYSIS database, our tool offers high-accuracy predictions, reflecting the latest in chemical research and data.
Strategy Settings
Precursor scoring | Relevance Heuristic |
---|---|
Min. plausibility | 0.01 |
Model | Template_relevance |
Template Set | Pistachio/Bkms_metabolic/Pistachio_ringbreaker/Reaxys/Reaxys_biocatalysis |
Top-N result to add to graph | 6 |
Feasible Synthetic Routes
Disclaimer and Information on In-Vitro Research Products
Please be aware that all articles and product information presented on BenchChem are intended solely for informational purposes. The products available for purchase on BenchChem are specifically designed for in-vitro studies, which are conducted outside of living organisms. In-vitro studies, derived from the Latin term "in glass," involve experiments performed in controlled laboratory settings using cells or tissues. It is important to note that these products are not categorized as medicines or drugs, and they have not received approval from the FDA for the prevention, treatment, or cure of any medical condition, ailment, or disease. We must emphasize that any form of bodily introduction of these products into humans or animals is strictly prohibited by law. It is essential to adhere to these guidelines to ensure compliance with legal and ethical standards in research and experimentation.