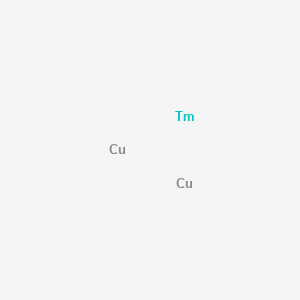
Copper;thulium
- Click on QUICK INQUIRY to receive a quote from our team of experts.
- With the quality product at a COMPETITIVE price, you can focus more on your research.
Overview
Description
Copper;thulium is a compound formed by the combination of copper and thulium Thulium is a rare earth element with the atomic number 69 and is part of the lanthanide series Copper, on the other hand, is a well-known transition metal with the atomic number 29
Preparation Methods
Synthetic Routes and Reaction Conditions: The preparation of copper;thulium involves several steps. One common method is the chemical reduction approach. For instance, copper nanoparticles can be synthesized by reducing aqueous copper salt using ascorbic acid as a reducing agent at low temperatures (around 80°C) . Thulium, being a rare earth element, is typically extracted from minerals such as monazite and xenotime sands . The calciothermic process is often used for the preparation of high-purity thulium .
Industrial Production Methods: Industrial production of this compound involves the combination of copper and thulium in controlled environments to ensure the purity and stability of the compound. The process may include the use of high-temperature furnaces and inert atmospheres to prevent oxidation and contamination.
Chemical Reactions Analysis
Types of Reactions: Copper;thulium undergoes various chemical reactions, including oxidation, reduction, and substitution. Thulium reacts with oxygen to form thulium(III) oxide, and with halogens to form halides . Copper, on the other hand, can react with carbonates to form copper(II) carbonate .
Common Reagents and Conditions:
Oxidation: Thulium reacts with oxygen at elevated temperatures to form thulium(III) oxide.
Reduction: Copper can be reduced from its salts using reducing agents like ascorbic acid.
Substitution: Thulium can react with halogens such as chlorine, bromine, and iodine to form respective halides.
Major Products Formed:
- Thulium(III) oxide (Tm2O3)
- Copper(II) carbonate (CuCO3)
- Thulium halides (TmX3, where X = F, Cl, Br, I)
Scientific Research Applications
Copper;thulium has several scientific research applications due to its unique properties:
Chemistry: Used in the synthesis of various compounds and as a catalyst in chemical reactions.
Biology: Thulium-doped fiber lasers are used in medical applications, including soft-tissue surgery.
Medicine: Thulium isotopes are used in portable X-ray devices.
Mechanism of Action
The mechanism of action of copper;thulium involves the interaction of copper and thulium ions with various molecular targets. Copper ions can act as a spermicide by increasing the levels of copper ions, prostaglandins, and white blood cells within the uterine and tubal fluids . Thulium ions, on the other hand, can interfere with steroid hormone receptors, inhibiting their biological effects .
Comparison with Similar Compounds
Thulium Compounds: Thulium reacts with various elements to form compounds such as thulium nitride (TmN), thulium sulfide (TmS), and thulium carbide (TmC2).
Copper Compounds: Copper forms compounds like copper(II) oxide (CuO), copper(I) oxide (Cu2O), and copper sulfate (CuSO4).
Uniqueness: Copper;thulium is unique due to the combination of properties from both copper and thulium. While copper is known for its excellent electrical conductivity and catalytic properties, thulium adds specialized applications in medical and industrial fields. The combination results in a compound with diverse applications and enhanced properties.
Properties
CAS No. |
60862-01-3 |
---|---|
Molecular Formula |
Cu2Tm |
Molecular Weight |
296.03 g/mol |
IUPAC Name |
copper;thulium |
InChI |
InChI=1S/2Cu.Tm |
InChI Key |
IPAIPPCEWCOTJG-UHFFFAOYSA-N |
Canonical SMILES |
[Cu].[Cu].[Tm] |
Origin of Product |
United States |
Disclaimer and Information on In-Vitro Research Products
Please be aware that all articles and product information presented on BenchChem are intended solely for informational purposes. The products available for purchase on BenchChem are specifically designed for in-vitro studies, which are conducted outside of living organisms. In-vitro studies, derived from the Latin term "in glass," involve experiments performed in controlled laboratory settings using cells or tissues. It is important to note that these products are not categorized as medicines or drugs, and they have not received approval from the FDA for the prevention, treatment, or cure of any medical condition, ailment, or disease. We must emphasize that any form of bodily introduction of these products into humans or animals is strictly prohibited by law. It is essential to adhere to these guidelines to ensure compliance with legal and ethical standards in research and experimentation.