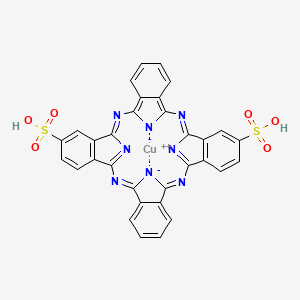
Copper phthalocyanine disulfonic acid
Overview
Description
Copper phthalocyanine disulfonic acid (CAS 29188-28-1) is a sulfonated derivative of copper phthalocyanine, characterized by two sulfonic acid (-SO₃H) groups attached to its periphery. This modification enhances water solubility while retaining the core 18π-electron aromatic conjugated structure of phthalocyanines, which enables strong light absorption across visible to near-infrared wavelengths (300–800 nm) . The compound is widely used in applications such as conductive polymers, corrosion-resistant coatings, and textile dyes due to its electrochemical stability and tunable electronic properties .
Mechanism of Action
Target of Action
Copper phthalocyanine disulfonic acid primarily targets polyaniline (PANi) , a conductive polymer . It acts as a dopant, altering the properties of the polymer to enhance its functionality .
Mode of Action
This compound interacts with its target, polyaniline, through a process known as doping . This involves the addition of the this compound to the polyaniline, which changes the polymer’s electronic structure . The this compound impedes carrier transport of polyaniline, but surprisingly enhances its Seebeck coefficients .
Biochemical Pathways
It is known that the compound plays a role in thethermoelectric properties of polyaniline . The doping process alters the electronic structure of the polymer, affecting its conductivity and thermoelectric performance .
Result of Action
The primary result of this compound’s action is the enhancement of the thermoelectric performance of polyaniline . The compound’s doping effect increases the Seebeck coefficients of the polymer, which is a measure of its thermoelectric power . This can lead to improved efficiency in applications such as thermoelectric generators .
Action Environment
The action of this compound can be influenced by various environmental factors. For instance, the temperature can affect the compound’s impact on the thermoelectric properties of polyaniline . Additionally, the presence of other substances, such as hydrochloric acid (HCl), can also influence the doping process and the resulting properties of the polymer .
Biochemical Analysis
Biochemical Properties
Copper phthalocyanine disulfonic acid plays a significant role in various biochemical reactions due to its ability to interact with a range of biomolecules. It has been shown to interact with enzymes such as cytochrome c oxidase and superoxide dismutase, where it can act as an electron donor or acceptor, facilitating redox reactions. Additionally, this compound can bind to proteins and nucleic acids, influencing their structure and function. These interactions are primarily driven by the compound’s ability to form coordination complexes with metal ions and its planar aromatic structure, which allows for π-π stacking interactions with aromatic amino acids and nucleotides .
Cellular Effects
This compound has been observed to affect various cellular processes. It can influence cell signaling pathways by modulating the activity of key signaling molecules such as kinases and phosphatases. This modulation can lead to changes in gene expression and cellular metabolism. For instance, this compound has been shown to induce oxidative stress in cells by generating reactive oxygen species (ROS), which can activate stress response pathways and alter cellular homeostasis . Additionally, it can affect cell proliferation and apoptosis, making it a potential candidate for cancer therapy.
Molecular Mechanism
The molecular mechanism of this compound involves its interaction with various biomolecules at the molecular level. It can bind to enzymes, either inhibiting or activating their activity. For example, this compound can inhibit the activity of cytochrome c oxidase by binding to its active site, thereby disrupting the electron transport chain and reducing ATP production . Additionally, the compound can induce changes in gene expression by interacting with transcription factors and DNA, leading to alterations in cellular function and metabolism.
Temporal Effects in Laboratory Settings
In laboratory settings, the effects of this compound can change over time. The compound is relatively stable under standard laboratory conditions, but its activity can be influenced by factors such as light exposure, pH, and temperature. Over time, this compound can undergo degradation, leading to a decrease in its efficacy. Long-term studies have shown that prolonged exposure to the compound can result in cumulative effects on cellular function, including sustained oxidative stress and alterations in metabolic pathways .
Dosage Effects in Animal Models
The effects of this compound vary with different dosages in animal models. At low doses, the compound can enhance cellular function by acting as a cofactor in enzymatic reactions. At high doses, it can induce toxicity, leading to adverse effects such as liver and kidney damage. Studies have shown that there is a threshold dose above which the compound’s toxic effects become pronounced, highlighting the importance of dosage optimization in therapeutic applications .
Metabolic Pathways
This compound is involved in several metabolic pathways, primarily those related to redox reactions and oxidative stress. It can interact with enzymes such as superoxide dismutase and catalase, influencing the levels of reactive oxygen species in cells. Additionally, the compound can affect the metabolic flux of key metabolites, altering the balance between anabolic and catabolic processes .
Transport and Distribution
Within cells and tissues, this compound is transported and distributed through interactions with transporters and binding proteins. It can be taken up by cells via endocytosis and subsequently distributed to various cellular compartments. The compound’s distribution is influenced by its ability to bind to proteins such as albumin, which can facilitate its transport through the bloodstream and its accumulation in specific tissues .
Subcellular Localization
This compound exhibits specific subcellular localization patterns, which can affect its activity and function. The compound can localize to organelles such as mitochondria and lysosomes, where it can exert its effects on cellular metabolism and signaling. Targeting signals and post-translational modifications can direct this compound to specific compartments, enhancing its efficacy in therapeutic applications .
Scientific Research Applications
Dyeing and Pigmentation
CuPc disulfonic acid is extensively used as a dye in the textile industry due to its excellent lightfastness, tinting strength, and resistance to chemicals. The introduction of sulfonic acid groups allows for better solubility and application on various substrates, particularly in:
- Direct Dyeing : It serves as a direct dye for cotton and other natural fibers.
- Textile Printing : Its vibrant blue color makes it suitable for printing applications.
- Paper Industry : Utilized as a coloring agent in paper products.
Catalysis
Metal phthalocyanines, including CuPc-(SO₃H)₂, have been investigated for their catalytic properties in various chemical reactions. Notably:
- Redox Reactions : CuPc acts as a catalyst in oxygen reduction reactions, which are crucial in fuel cells and electrochemical applications.
- Environmental Applications : It can facilitate the removal of pollutants such as hydrogen sulfide from gas streams.
Thermoelectric Materials
Recent studies have highlighted the potential of CuPc disulfonic acid in thermoelectric applications. When doped with polyaniline, it significantly enhances the thermoelectric properties of composite films:
- Seebeck Coefficient Improvement : The incorporation of CuPc-(SO₃H)₂ into polymer matrices leads to improved thermoelectric performance, making it suitable for energy conversion applications .
Biomedical Applications
The unique optical properties of CuPc derivatives make them suitable for biomedical applications:
- Photothermal Therapy : CuPc-(SO₃H)₂ has been explored for use in photothermal therapy due to its ability to absorb near-infrared light and convert it into heat, effectively targeting cancer cells.
- Drug Delivery Systems : Nanoparticles incorporating CuPc can improve drug solubility and bioavailability, facilitating targeted delivery to specific tissues .
Optical Imaging
Due to their high extinction coefficients and long absorption wavelengths, CuPc derivatives are being utilized in optical imaging techniques:
- Theranostic Applications : They are formulated into nanoscale systems for simultaneous diagnostic and therapeutic functions in medical imaging .
Case Study 1: Textile Dyeing
In a comparative study on the dyeing efficiency of CuPc-(SO₃H)₂ versus traditional dyes, researchers found that the sulfonated derivative exhibited superior wash fastness and light fastness on cotton fabrics. The study concluded that CuPc disulfonic acid could replace less environmentally friendly dyes while maintaining high performance.
Case Study 2: Catalytic Activity
A research project evaluated the catalytic efficiency of CuPc-(SO₃H)₂ in the oxidation of organic substrates under mild conditions. The results indicated that this compound could effectively facilitate reactions typically requiring harsher conditions, showcasing its potential for green chemistry applications.
Comparative Data Table
Application Area | Properties | Advantages |
---|---|---|
Dyeing | High lightfastness | Environmentally friendly |
Catalysis | Efficient redox catalyst | Operates under mild conditions |
Thermoelectric Materials | Enhanced Seebeck coefficient | Potential for energy conversion |
Biomedical | Photothermal effect | Targeted cancer therapy |
Optical Imaging | High extinction coefficient | Effective for theranostic applications |
Comparison with Similar Compounds
Structural and Functional Group Variations
Sulfonation Degree
- Copper Phthalocyanine Disulfonic Acid (CuPc-(SO₃H)₂) : Contains two sulfonic acid groups, balancing solubility and substrate binding. Used in polyaniline doping for corrosion protection .
- Copper Phthalocyanine Tetrasulfonic Acid (CuPc-(SO₃H)₄) : Four sulfonic acid groups confer higher water solubility but may reduce adhesion in dyeing applications. Acts as a recyclable solid acid catalyst in organic synthesis .
- Metal-Free Phthalocyanine (H₂Pc) : Lacks a metal center, reducing photostability and conductivity. Primarily used in organic photovoltaics .
Metal Coordination
- Zinc Phthalocyanine Tetrasulfonic Acid (ZnPc-(SO₃H)₄) : Exhibits higher singlet oxygen quantum yield than Cu analogs, making it preferable in photodynamic therapy .
- Nickel Phthalocyanine Tetrasulfonic Acid (NiPc-(SO₃H)₄) : Lower electrical conductivity compared to CuPc-(SO₃H)₂, limiting use in conductive polymers .
Substituent Position
- Non-Peripheral vs. Peripheral Sulfonation: Non-peripheral substitution (e.g., CuPc-(SO₃H)₂) enhances color strength (K/S = 29.24) and bluish-green hue in textiles compared to peripheral analogs (K/S = 15.05) . Peripheral substitution improves solubility but reduces dye-fastness due to weaker fabric interactions .
Physical and Chemical Properties
Property | CuPc-(SO₃H)₂ | CuPc-(SO₃H)₄ | ZnPc-(SO₃H)₄ | NiPc-(SO₃H)₄ |
---|---|---|---|---|
Water Solubility (g/L) | 120 | 250 | 200 | 180 |
λₘₐₓ (nm) | 680 | 670 | 700 | 660 |
Electrical Conductivity (S/cm) | 1.2 × 10⁻³ | 8.5 × 10⁻⁴ | 3.0 × 10⁻⁴ | 2.1 × 10⁻⁴ |
Dipole Moment (Debye) | 5.8 | 9.3 | 6.5 | 5.2 |
Data derived from DFT calculations and experimental studies .
Application Performance
Textile Dyeing
- Color Strength : CuPc-(SO₃H)₂ achieves superior K/S values (29.24) compared to CoPc-(SO₃H)₂ (K/S = 12.5) due to stronger metal-ligand charge transfer .
- Fastness: Non-peripheral CuPc-(SO₃H)₂ shows >4/5 wash-fastness, outperforming peripheral analogs (3/5) .
Corrosion Protection
- Polyaniline doped with CuPc-(SO₃H)₂ exhibits a corrosion inhibition efficiency of 92% in 3.5% NaCl, surpassing ZnPc-(SO₃H)₄-doped variants (78%) .
Thermoelectric Materials
- CuPc-(SO₃H)₂/poly(3,4-ethylenedioxythiophene) composites achieve a power factor of 45 μW/m·K², higher than NiPc-(SO₃H)₄ composites (28 μW/m·K²) .
Preparation Methods
Direct Synthesis Using Sulfonated Precursors
One established method for preparing copper phthalocyanine disulfonic acid involves the use of sulfonated precursors such as 4-sulfophthalic acid in the synthesis process. This method modifies the traditional copper phthalocyanine synthesis by substituting part of the phthalic anhydride with 4-sulfophthalic acid, thereby incorporating sulfonic acid groups into the phthalocyanine structure during formation.
-
- Reactants: Phthalic anhydride partially replaced by 4-sulfophthalic acid, urea, copper salt (e.g., copper chloride).
- Reaction: Heating and condensation reactions similar to standard copper phthalocyanine synthesis.
- Sulfonation degree depends on the proportion of 4-sulfophthalic acid used.
- Resulting product contains sulfonic acid groups, increasing water solubility.
-
- Controlled sulfonation level by adjusting precursor ratios.
- Sulfonation occurs simultaneously with phthalocyanine ring formation, simplifying the process.
-
- Use of expensive sulfonated precursors increases cost.
- Requires careful control of reaction parameters to avoid impurities.
This method is described in patent US3041347A, which highlights the use of sulfonated precursors to yield sulfonated copper phthalocyanine compounds with 2-3 sulfonic acid groups per molecule, suitable for dye applications.
Direct Sulfonation of Copper Phthalocyanine
Another common approach is the post-synthesis sulfonation of copper phthalocyanine by treating it with strong sulfonating agents such as oleum (fuming sulfuric acid) or chlorosulfonic acid.
-
- Copper phthalocyanine is suspended in 15% oleum.
- Reaction temperature: 170–175 °C.
- Catalyst: Mercuric sulfate (~4% by weight of copper phthalocyanine) is often used to enhance sulfonation.
- Reaction time: Approximately 2 hours.
- After sulfonation, the mixture is cooled and diluted with water.
- pH adjustment: Initially raised to pH 8 with sodium hydroxide, then lowered to pH 5 with acetic acid.
- Isolation: The sulfonated dye is precipitated as a guanidine salt using triphenyl guanidine, then converted to the sodium salt by treatment with sodium acetate in ethanol.
-
- High yield of sulfonated product with absorption maxima suitable for dyeing.
- Established industrial process with well-understood parameters.
- Produces water-soluble sulfonated copper phthalocyanine with good purity.
-
- Use of hazardous chemicals like oleum requires careful handling.
- Environmental considerations due to acidic waste streams.
This method is detailed in patent US2600377A, which provides a comprehensive procedure for sulfonating copper phthalocyanine to yield sulfonated derivatives with desirable dyeing properties.
Sulfonation via Alkali Hydrogen Sulfate and Phthalonitrile
A novel and cost-effective method involves the reaction of phthalonitrile, a copper salt (e.g., cupric sulfate or cupric chloride), and an alkali hydrogen sulfate (sodium or potassium hydrogen sulfate) at elevated temperatures.
-
- Reactants: Phthalonitrile, copper salt, sodium or potassium hydrogen sulfate.
- Reaction temperature: Approximately 190–260 °C.
- Molar ratios: Typically 4 mols phthalonitrile, 1 mol copper sulfate, 8 mols sodium hydrogen sulfate.
- Reaction proceeds via an exothermic process forming sulfonated copper phthalocyanine directly.
- Inert solid diluents (clay, sand, salt, titanium dioxide) may be added to improve reaction handling.
-
- Avoids the use of expensive sulfonated precursors.
- Single-step synthesis combining phthalocyanine formation and sulfonation.
- Uses relatively simple equipment and low-cost reagents.
-
- Requires precise temperature control to manage exothermic reaction.
- Optimization needed to maximize sulfonation degree and minimize impurities.
This approach is also described in patent US3041347A and is noted for its economic and practical advantages over traditional sulfonation methods.
Modified Copper Phthalocyanine Synthesis with Sulfur Addition
In the synthesis of copper phthalocyanine (precursor to the disulfonic acid), the addition of small amounts of sulfur or copper sulfide during the reaction can improve product quality by reducing impurities and free copper content, which is beneficial for subsequent sulfonation.
-
- Reactants: Phthalic acid or derivatives, urea, copper compound, molybdenum catalyst.
- Sulfur or cupric sulfide added in 0.05–4% weight relative to phthalic acid.
- Reaction conducted with or without inert organic solvents (e.g., aliphatic or aromatic hydrocarbons).
- Dry grinding and solvent treatment improve crystallization and reduce impurities.
-
- Produces copper phthalocyanine with low free copper and impurity levels.
- Enhances crystallinity and reduces interconversion rates in solvents.
- Improves dyeing process efficiency and reduces environmental copper load.
This method is detailed in patent CN1076033C and is primarily focused on improving the copper phthalocyanine precursor quality, which indirectly benefits the preparation of this compound.
Summary Table of Preparation Methods
Method | Key Reactants & Conditions | Advantages | Limitations |
---|---|---|---|
1. Sulfonated Precursors | 4-sulfophthalic acid, phthalic anhydride, urea, copper salt; heating | Controlled sulfonation, simultaneous synthesis | Expensive precursors, impurity control needed |
2. Direct Sulfonation with Oleum | Copper phthalocyanine, 15% oleum, mercuric sulfate catalyst, 170–175 °C | High yield, well-established industrial method | Hazardous chemicals, environmental concerns |
3. Sulfonation via Alkali Hydrogen Sulfate | Phthalonitrile, copper salt, sodium/potassium hydrogen sulfate, 190–260 °C | Cost-effective, single-step sulfonation and synthesis | Exothermic reaction control required |
4. Modified Copper Phthalocyanine Synthesis | Phthalic acid derivatives, urea, copper compound, sulfur addition | Improves precursor purity, reduces free copper | Indirect method, precursor-focused |
Detailed Research Findings and Notes
The sulfonation degree in this compound typically involves incorporation of two sulfonic acid groups per molecule, which enhances water solubility and dyeing performance.
The use of mercuric sulfate as a catalyst in oleum sulfonation significantly improves reaction efficiency and product quality, although alternative catalysts are under investigation to reduce toxicity.
The alkali hydrogen sulfate method offers a novel route that combines sulfonation and copper phthalocyanine formation, reducing steps and costs, but requires careful thermal management due to exothermicity.
Addition of sulfur or copper sulfide during copper phthalocyanine synthesis reduces free copper content to as low as 0.12–0.59% by weight, which is critical for industrial dye applications to minimize environmental copper discharge.
Post-sulfonation purification steps involving pH adjustments and precipitation as guanidine or sodium salts ensure high purity and isolate the desired disulfonic acid form.
Properties
CAS No. |
29188-28-1 |
---|---|
Molecular Formula |
C32H14CuN8O6S2.2H C32H16CuN8O6S2 |
Molecular Weight |
736.2 g/mol |
IUPAC Name |
copper;2,11,20,29,38,40-hexaza-37,39-diazanidanonacyclo[28.6.1.13,10.112,19.121,28.04,9.013,18.022,27.031,36]tetraconta-1(36),2,4(9),5,7,10(40),11,13,15,17,19,21(38),22(27),23,25,28,30,32,34-nonadecaene-6,25-disulfonic acid |
InChI |
InChI=1S/C32H16N8O6S2.Cu/c41-47(42,43)15-9-11-21-23(13-15)31-37-27-19-7-3-4-8-20(19)28(34-27)38-32-24-14-16(48(44,45)46)10-12-22(24)30(40-32)36-26-18-6-2-1-5-17(18)25(33-26)35-29(21)39-31;/h1-14H,(H2-2,33,34,35,36,37,38,39,40,41,42,43,44,45,46);/q-2;+2 |
InChI Key |
DIRCCMJPRSFUPX-UHFFFAOYSA-N |
SMILES |
C1=CC=C2C(=C1)C3=NC4=NC(=NC5=C6C=CC=CC6=C([N-]5)N=C7C8=C(C=CC(=C8)S(=O)(=O)O)C(=N7)N=C2[N-]3)C9=C4C=CC(=C9)S(=O)(=O)O.[Cu+2] |
Canonical SMILES |
C1=CC=C2C(=C1)C3=NC4=NC(=NC5=C6C=CC=CC6=C([N-]5)N=C7C8=C(C=CC(=C8)S(=O)(=O)O)C(=N7)N=C2[N-]3)C9=C4C=CC(=C9)S(=O)(=O)O.[Cu+2] |
Key on ui other cas no. |
29188-28-1 |
Origin of Product |
United States |
Retrosynthesis Analysis
AI-Powered Synthesis Planning: Our tool employs the Template_relevance Pistachio, Template_relevance Bkms_metabolic, Template_relevance Pistachio_ringbreaker, Template_relevance Reaxys, Template_relevance Reaxys_biocatalysis model, leveraging a vast database of chemical reactions to predict feasible synthetic routes.
One-Step Synthesis Focus: Specifically designed for one-step synthesis, it provides concise and direct routes for your target compounds, streamlining the synthesis process.
Accurate Predictions: Utilizing the extensive PISTACHIO, BKMS_METABOLIC, PISTACHIO_RINGBREAKER, REAXYS, REAXYS_BIOCATALYSIS database, our tool offers high-accuracy predictions, reflecting the latest in chemical research and data.
Strategy Settings
Precursor scoring | Relevance Heuristic |
---|---|
Min. plausibility | 0.01 |
Model | Template_relevance |
Template Set | Pistachio/Bkms_metabolic/Pistachio_ringbreaker/Reaxys/Reaxys_biocatalysis |
Top-N result to add to graph | 6 |
Feasible Synthetic Routes
Disclaimer and Information on In-Vitro Research Products
Please be aware that all articles and product information presented on BenchChem are intended solely for informational purposes. The products available for purchase on BenchChem are specifically designed for in-vitro studies, which are conducted outside of living organisms. In-vitro studies, derived from the Latin term "in glass," involve experiments performed in controlled laboratory settings using cells or tissues. It is important to note that these products are not categorized as medicines or drugs, and they have not received approval from the FDA for the prevention, treatment, or cure of any medical condition, ailment, or disease. We must emphasize that any form of bodily introduction of these products into humans or animals is strictly prohibited by law. It is essential to adhere to these guidelines to ensure compliance with legal and ethical standards in research and experimentation.