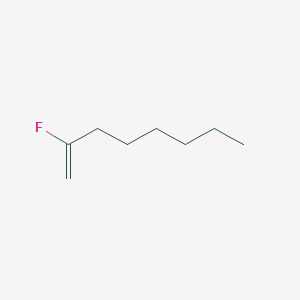
2-Fluorooct-1-ene
- Click on QUICK INQUIRY to receive a quote from our team of experts.
- With the quality product at a COMPETITIVE price, you can focus more on your research.
Overview
Description
2-Fluorooct-1-ene is an organic compound that belongs to the class of fluoroalkenes It is characterized by the presence of a fluorine atom attached to the second carbon of an octene chain
Preparation Methods
Synthetic Routes and Reaction Conditions: The synthesis of 2-Fluorooct-1-ene typically involves the fluorination of oct-1-ene. One common method is the addition of hydrogen fluoride (HF) to oct-1-ene under controlled conditions. This reaction requires a catalyst, such as antimony pentachloride (SbCl5), to facilitate the addition of the fluorine atom to the desired position on the carbon chain.
Industrial Production Methods: Industrial production of this compound can be achieved through large-scale fluorination processes. These processes often involve the use of specialized reactors that can handle the corrosive nature of hydrogen fluoride. The reaction conditions, including temperature and pressure, are optimized to maximize yield and purity of the final product.
Chemical Reactions Analysis
Types of Reactions: 2-Fluorooct-1-ene undergoes various types of chemical reactions, including:
Substitution Reactions: The fluorine atom can be substituted by other nucleophiles, such as hydroxide ions (OH-) or amines.
Addition Reactions: The double bond in this compound can participate in addition reactions with halogens, hydrogen, or other electrophiles.
Oxidation Reactions: The compound can be oxidized to form fluorinated alcohols or ketones.
Common Reagents and Conditions:
Substitution Reactions: Typically carried out in the presence of a strong base, such as sodium hydroxide (NaOH), at elevated temperatures.
Addition Reactions: Often performed using halogens like bromine (Br2) or hydrogen gas (H2) in the presence of a catalyst.
Oxidation Reactions: Commonly use oxidizing agents like potassium permanganate (KMnO4) or chromium trioxide (CrO3).
Major Products:
Substitution Reactions: Fluorinated alcohols or amines.
Addition Reactions: Dihalogenated or hydrogenated products.
Oxidation Reactions: Fluorinated ketones or carboxylic acids.
Scientific Research Applications
2-Fluorooct-1-ene has a wide range of applications in scientific research:
Chemistry: Used as a building block in the synthesis of more complex fluorinated compounds. Its unique reactivity makes it valuable in the development of new synthetic methodologies.
Biology: Investigated for its potential use in the modification of biomolecules, such as proteins and nucleic acids, to study their structure and function.
Medicine: Explored for its potential in drug development, particularly in the design of fluorinated pharmaceuticals with improved metabolic stability and bioavailability.
Industry: Utilized in the production of specialty polymers and materials with enhanced chemical resistance and thermal stability.
Mechanism of Action
The mechanism of action of 2-Fluorooct-1-ene involves its interaction with various molecular targets. The presence of the fluorine atom significantly alters the electronic properties of the compound, making it more reactive towards nucleophiles and electrophiles. This reactivity is exploited in various chemical transformations, where this compound acts as an intermediate or a reactant.
Molecular Targets and Pathways:
Nucleophilic Attack: The fluorine atom’s electronegativity makes the adjacent carbon more susceptible to nucleophilic attack.
Electrophilic Addition: The double bond in this compound can undergo electrophilic addition reactions, forming new carbon-fluorine bonds.
Comparison with Similar Compounds
2-Fluorooct-1-ene can be compared with other fluoroalkenes, such as:
2-Fluorobut-1-ene: A shorter chain fluoroalkene with similar reactivity but different physical properties.
2-Fluorohex-1-ene: An intermediate chain fluoroalkene with properties between those of 2-Fluorobut-1-ene and this compound.
2-Fluorodec-1-ene: A longer chain fluoroalkene with increased hydrophobicity and different reactivity patterns.
Uniqueness: The uniqueness of this compound lies in its optimal chain length, which provides a balance between reactivity and physical properties. This makes it a versatile compound for various applications in organic synthesis and material science.
Biological Activity
2-Fluorooct-1-ene is a fluorinated organic compound that has garnered interest in medicinal chemistry and materials science due to its unique biological activities and potential applications. This article delves into the biological activity of this compound, focusing on its pharmacological effects, mechanisms of action, and relevant case studies.
This compound is characterized by its fluorine substitution at the second carbon of an octene chain. The presence of fluorine significantly alters the compound's reactivity and biological interactions compared to its non-fluorinated counterparts.
Biological Activity Overview
The biological activity of this compound has been explored in various contexts, including its effects on cancer cells, antimicrobial properties, and potential as a therapeutic agent.
Anticancer Activity
Research indicates that fluorinated compounds can exhibit potent anticancer properties. In particular, studies have shown that this compound derivatives can inhibit glycolytic enzymes, which are often upregulated in aggressive cancers like glioblastoma multiforme (GBM). This inhibition can lead to reduced cancer cell proliferation and enhanced apoptosis.
Table 1: Summary of Anticancer Activity Studies on Fluorinated Compounds
Antimicrobial Activity
In addition to its anticancer effects, this compound has demonstrated antimicrobial properties. Research has shown that fluorinated compounds can disrupt bacterial cell membranes and inhibit essential metabolic pathways.
Table 2: Antimicrobial Activity of Fluorinated Compounds
Case Study 1: Anticancer Efficacy in GBM
A study investigated the efficacy of various fluorinated analogs, including this compound, in inhibiting GBM cell growth. The results indicated that these compounds significantly reduced cell viability through the modulation of glycolytic pathways. The study highlighted the potential for developing new treatments targeting cancer metabolism.
Case Study 2: Antimicrobial Properties
Another study evaluated the antimicrobial effects of this compound against a range of pathogens. The compound exhibited significant activity against Gram-positive bacteria, suggesting its potential as a lead compound for developing new antibiotics.
The mechanisms underlying the biological activities of this compound are multifaceted:
- Enzyme Inhibition : The fluorine atom enhances the binding affinity to target enzymes such as hexokinase, leading to effective inhibition.
- Membrane Interaction : The lipophilic nature of fluorinated compounds allows them to integrate into bacterial membranes, disrupting their integrity.
- Metabolic Modulation : By altering metabolic pathways, particularly glycolysis, these compounds can shift cellular energy dynamics, promoting apoptosis in cancer cells.
Properties
CAS No. |
61350-08-1 |
---|---|
Molecular Formula |
C8H15F |
Molecular Weight |
130.20 g/mol |
IUPAC Name |
2-fluorooct-1-ene |
InChI |
InChI=1S/C8H15F/c1-3-4-5-6-7-8(2)9/h2-7H2,1H3 |
InChI Key |
GQQFAFOCRSCFCS-UHFFFAOYSA-N |
Canonical SMILES |
CCCCCCC(=C)F |
Origin of Product |
United States |
Disclaimer and Information on In-Vitro Research Products
Please be aware that all articles and product information presented on BenchChem are intended solely for informational purposes. The products available for purchase on BenchChem are specifically designed for in-vitro studies, which are conducted outside of living organisms. In-vitro studies, derived from the Latin term "in glass," involve experiments performed in controlled laboratory settings using cells or tissues. It is important to note that these products are not categorized as medicines or drugs, and they have not received approval from the FDA for the prevention, treatment, or cure of any medical condition, ailment, or disease. We must emphasize that any form of bodily introduction of these products into humans or animals is strictly prohibited by law. It is essential to adhere to these guidelines to ensure compliance with legal and ethical standards in research and experimentation.