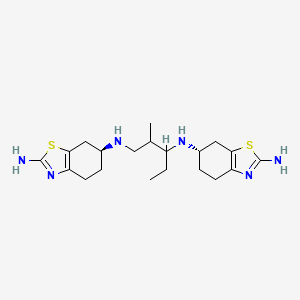
Pramipexole dimer
Overview
Description
Pramipexole dimer is a degradation impurity identified during the synthesis or storage of pramipexole dihydrochloride, a non-ergoline dopamine agonist used primarily for Parkinson’s disease (PD) and restless legs syndrome (RLS) . Structurally, it arises from the covalent linkage of two pramipexole molecules, forming a dimeric byproduct. This impurity is pharmacopeially recognized as "Impurity C" in the United States Pharmacopeia (USP) and European Pharmacopeia (Ph. Eur.) . Its isolation and characterization are critical for quality control, as regulatory standards mandate strict limits (typically ≤0.15%) for such impurities in drug formulations .
Preparation Methods
Synthetic Routes for Pramipexole Dimer
The this compound is formed by the covalent linkage of two pramipexole molecules, typically arising as an impurity during synthesis or storage. The core synthetic step involves the reaction of the key intermediate (S)-2,6-diamino-4,5,6,7-tetrahydrobenzothiazole with an alkylating agent under controlled conditions.
- Key Reaction: The dimerization occurs via alkylation where two pramipexole units are linked covalently, often through nitrogen atoms on the diamino benzothiazole ring system.
- Reaction Conditions: The reaction is generally conducted in the absence of a base to favor selective N-monoalkylation, which leads to the precipitation of the dimeric product as a salt from the solvent system used.
- Solvent Selection: Solvents are chosen to promote selective precipitation and facilitate isolation of the dimer. Common solvents include organic solvents compatible with the alkylating agent and pramipexole intermediate.
This synthetic route is favored for its simplicity and ability to isolate the dimer as a solid salt, facilitating purification and characterization.
Industrial Scale Preparation and Process Optimization
Industrial production methods for pramipexole and its dimers focus on maximizing yield and purity while controlling impurity formation.
- Starting Materials: The synthesis begins with the compound of formula II (a precursor) which undergoes bromination and thiourea treatment to yield an intermediate (compound of formula III).
- Reduction Step: The intermediate is reduced using borane-tetrahydrofuran complex under nitrogen atmosphere at controlled temperatures (35°C to 60°C), followed by acidification and extraction steps to isolate the racemic pramipexole compound.
- Resolution: The racemic mixture is resolved using chiral auxiliaries to obtain the active (-) isomer.
- Dimer Formation Control: During these steps, conditions such as temperature, solvent choice, and reaction time are optimized to minimize dimer formation as an impurity.
- Purification: Crystallization techniques, including the use of ethanol and ethyl acetate, are employed to isolate pure pramipexole and reduce dimer content.
The process involves careful quenching of reagents, filtration, washing, and drying steps to ensure the final product meets pharmacopeial standards.
Purification and Isolation Techniques
Purification is crucial to separate this compound from the main product and other impurities:
Step | Description |
---|---|
Filtration and Washing | After reaction completion, solids are filtered and washed with solvents like ethyl acetate or methanol to remove impurities. |
Crystallization | Controlled crystallization from solvents such as ethanol or ethyl acetate-sherwood oil mixtures to isolate pure dimer or pramipexole. |
pH Adjustment | After reaction, basification to pH 9-10 followed by extraction helps in purifying the compound. |
Drying | Air-drying or vacuum drying at controlled temperatures (25°C to 40°C) ensures removal of residual solvents and moisture. |
These steps ensure the isolation of this compound with high purity suitable for analytical reference or impurity profiling.
Reaction Parameters and Conditions
Parameter | Typical Conditions | Notes |
---|---|---|
Temperature | 0°C to 80°C (varies by step) | Bromination at ambient to 25°C; reduction at 35-60°C; crystallization at 10°C to -15°C |
Solvents | Tetrahydrofuran, diethyl ether, ethanol, ethyl acetate | Solvent choice critical for solubility and selective precipitation |
Reducing Agent | Borane-tetrahydrofuran complex | Used for reduction of intermediate compound III |
Reaction Time | 1 to 6 hours | Bromination 2-5 hours; thiourea reflux 1-4 hours |
Atmosphere | Nitrogen | To avoid oxidation and side reactions |
The control of these parameters is essential for reproducibility and minimizing side products such as the this compound.
Analytical and Quality Control Considerations
- The this compound is recognized pharmacopeially as "Impurity C" and must be controlled to ≤0.15% in drug substances.
- Analytical methods include thin-layer chromatography, HPLC, and gas chromatography for monitoring reaction completion and impurity levels.
- Characterization techniques such as X-ray diffraction and differential scanning calorimetry have been used to identify polymorphic forms of pramipexole salts, which may influence impurity profiles.
Summary Table: Preparation Methods Overview
Preparation Step | Description | Key Reagents/Conditions | Outcome |
---|---|---|---|
Bromination | Addition of bromine dropwise to precursor in organic solvent | Bromine, acetic acid, chloroform, THF | Formation of brominated intermediate |
Thiourea Treatment | Reflux with thiourea to convert brominated intermediate | Thiourea, reflux 1-4 hours | Intermediate compound III |
Reduction | Reduction with borane-THF complex under nitrogen atmosphere | Borane-THF, 35-60°C, nitrogen | Racemic pramipexole |
Resolution | Use of chiral auxiliaries to isolate (-) isomer | Chiral resolving agents | Pure (-) pramipexole |
Dimer Formation (Side Reaction) | Reaction of diamino benzothiazole with alkylating agent, absence of base favors dimer precipitation | Alkylating agent, no base, selective solvents | This compound (Impurity C) |
Purification and Isolation | Filtration, washing, crystallization, pH adjustment | Ethyl acetate, ethanol, pH 9-10 | High purity pramipexole and dimer |
Chemical Reactions Analysis
Types of Reactions
Pramipexole dimer undergoes various chemical reactions, including:
Oxidation: Involves the addition of oxygen or the removal of hydrogen.
Reduction: Involves the addition of hydrogen or the removal of oxygen.
Substitution: Involves the replacement of one functional group with another.
Common Reagents and Conditions
Common reagents used in these reactions include oxidizing agents like potassium permanganate, reducing agents like sodium borohydride, and various alkylating agents. The reactions are typically carried out under controlled conditions to ensure the desired product formation.
Major Products Formed
The major products formed from these reactions depend on the specific reagents and conditions used. For example, oxidation of this compound may result in the formation of corresponding oxides, while reduction may yield reduced amine derivatives.
Scientific Research Applications
Pharmacological Applications
2.1 Enhanced Therapeutic Effects
Research indicates that pramipexole dimer may exhibit improved binding affinity to dopamine receptors compared to its monomeric form. This enhanced interaction could result in more pronounced therapeutic effects, particularly in managing Parkinson's disease symptoms. Studies suggest that the dimer could potentially act on multiple receptor subtypes, thereby offering a broader therapeutic profile .
2.2 Drug Development
The this compound is essential in the development of new formulations aimed at improving bioavailability and reducing side effects associated with the monomer. Its role in Abbreviated New Drug Applications (ANDA) and New Drug Applications (NDA) is crucial for regulatory submissions and commercial production .
Analytical Applications
3.1 Electrochemical Detection Methods
Recent studies have focused on developing sensitive electrochemical sensors for the detection of pramipexole and its derivatives, including the dimer. An electrochemical sensor utilizing graphitic carbon nitride (gCN) has been successfully developed to detect trace levels of pramipexole, demonstrating high sensitivity and selectivity . This method is significant for monitoring drug levels in biological samples, ensuring safe therapeutic use.
3.2 Identification of Degradation Products
The stability of pramipexole formulations is critical for ensuring efficacy. Research has identified degradation impurities related to drug-excipient interactions using high-performance liquid chromatography (HPLC) and nuclear magnetic resonance (NMR) techniques . Understanding these degradation pathways can inform the formulation process and enhance product stability.
Mechanism of Action
Pramipexole dimer exerts its effects by stimulating dopamine receptors in the brain, particularly the D2, D3, and D4 receptors . This stimulation helps alleviate symptoms associated with dopamine deficiency, such as those seen in Parkinson’s disease. The exact mechanism involves binding to these receptors and mimicking the action of dopamine, thereby improving motor function and reducing symptoms like tremors and rigidity .
Comparison with Similar Compounds
Structural and Pharmacological Differences
Table 1: Key Structural and Pharmacological Properties
Key Findings :
- This compound lacks pharmacological characterization but is structurally distinct due to dimerization, which likely abolishes dopamine receptor binding .
- CJ-1638 and D-264 , derived from pramipexole, exhibit enhanced D3 receptor selectivity but suffer from rapid hepatic metabolism, limiting clinical utility .
- N-Propylpramipexole (Impurity B) is another pharmacopeial impurity but differs in its alkylation pattern, which may alter pharmacokinetics .
Table 2: Stability and Formation Conditions
Key Findings :
- This compound forms under stress conditions (e.g., 40°C with mannitol), highlighting excipient compatibility challenges .
- In contrast, CJ-1638 and related analogs are intentionally synthesized for D3 selectivity but require structural optimization to improve metabolic stability .
Regulatory and Analytical Considerations
Table 3: Regulatory Limits and Analytical Standards
Key Findings :
Biological Activity
Pramipexole, a dopamine D2/D3 receptor agonist, is primarily used in the treatment of Parkinson's disease and restless legs syndrome. The compound has shown significant biological activity, particularly in neuroprotection, pain relief, and cognitive enhancement. This article explores the biological activity of pramipexole dimer through various studies and case reports, highlighting its mechanisms of action, efficacy, and potential applications.
Pramipexole acts predominantly on dopamine D2 and D3 receptors, exhibiting a higher affinity for D3 receptors. This selectivity is crucial for its therapeutic effects, particularly in neuroprotection and modulation of dopaminergic signaling.
- Dopamine Receptor Agonism : Pramipexole enhances dopaminergic transmission by stimulating D2/D3 receptors, which is beneficial in alleviating motor symptoms associated with Parkinson's disease .
- Neuroprotective Properties : Research indicates that pramipexole promotes neuronal survival through mitochondrial pathways. It reduces oxidative stress and mitochondrial dysfunction following ischemic events, thereby preventing cell death .
- Anti-inflammatory Effects : Pramipexole has been shown to modulate inflammatory responses in neurodegenerative conditions, contributing to its neuroprotective effects .
Case Studies
- Cognitive Impairment in Parkinson's Disease : A study involving 50 patients assessed the impact of pramipexole combined with nerve growth factor (NGF) on cognitive function. Results indicated significant improvements in cognitive performance and reductions in urinary biomarkers associated with Alzheimer's pathology .
- Chronic Pain Management : An 80-year-old male patient suffering from chronic low back pain and undiagnosed ADHD was treated with pramipexole alongside atomoxetine. This combination led to substantial improvements in pain management and cognitive function, demonstrating pramipexole's potential beyond traditional indications .
Observational Studies
A prospective observational study involving 657 Parkinson's disease patients highlighted pramipexole's effectiveness in improving not only motor symptoms but also depressive symptoms. The study utilized validated scales to measure outcomes related to daily living activities and psychological status .
Table 1: Summary of Efficacy Studies on Pramipexole
Q & A
Basic Research Questions
Q. What are the critical analytical techniques for confirming the structural integrity of Pramipexole dimer post-synthesis?
- Methodological Answer : Use high-performance liquid chromatography (HPLC) to assess purity (>98%) and nuclear magnetic resonance (NMR) spectroscopy to verify dimeric bonding. Cross-validate with mass spectrometry (MS) for molecular weight confirmation. Ensure consistency by comparing results with published reference spectra from peer-reviewed studies. For reproducibility, document solvent systems, column types, and instrument calibration protocols in detail .
数学研究员为数不多的物质积累 | 一场别出心裁的数学书籍测评17:22
Q. How can researchers standardize synthesis protocols for this compound to ensure cross-laboratory reproducibility?
- Methodological Answer : Develop step-by-step synthesis protocols with controlled parameters (e.g., reaction temperature, stoichiometric ratios, and catalyst purity). Use collaborative platforms like ResearchGate to share raw data and procedural videos. Validate batches via third-party laboratories using blinded sample testing to eliminate bias .
【10个免费文献下载网站】完胜知网的论文神器|论文狗必备|科研写作小帮手|科研必备工具|毕业论文写作工具05:45
Q. What in vitro assays are most suitable for preliminary evaluation of this compound’s dopamine receptor affinity?
- Methodological Answer : Radioligand binding assays (e.g., using ³H-Spiperone for D2/D3 receptors) under physiologically relevant pH and temperature conditions. Include negative controls (e.g., monomeric Pramipexole) and triplicate measurements to account for variability. Normalize data against reference agonists like Quinpirole .
正主来啦~2022.05孟德尔随机化操作。去年的第一次操作课程没有加密,被各种转发。因为是第一次授课,也有不少瑕疵以及很多内容没有讲到。重点还是看高质量答疑!2:56:25
Advanced Research Questions
Q. How should researchers design longitudinal studies to resolve contradictions in this compound’s neuroprotective efficacy across preclinical models?
- Methodological Answer : Implement a factorial design comparing dose-response curves in both rodent and non-rodent models (e.g., zebrafish). Use Bayesian statistical models to account for interspecies variability. Publish raw datasets with metadata (e.g., animal age, diet, and circadian cycle) to facilitate meta-analyses .
Q. What computational strategies optimize the prediction of this compound’s blood-brain barrier (BBB) permeability?
- Methodological Answer : Combine molecular dynamics simulations (e.g., using GROMACS) with in situ perfusion data. Adjust force-field parameters to reflect dimer-specific hydrophobicity. Validate predictions via in vivo microdialysis in rodent models, correlating computational outputs with experimental AUC (area under the curve) values .
Q. How can metabolic stability studies for this compound address discrepancies in cytochrome P450 (CYP) interaction data?
- Methodological Answer : Conduct time-dependent CYP inhibition assays (e.g., CYP3A4, CYP2D6) using human liver microsomes. Compare results with monomeric Pramipexole to isolate dimer-specific effects. Apply replicated analysis by repeating experiments in independent labs with standardized enzyme sources .
Q. What experimental frameworks are recommended for elucidating this compound’s off-target effects in neurodegenerative disease models?
- Methodological Answer : Use transcriptomic profiling (RNA-seq) of treated vs. untreated neuronal cultures. Pair with cheminformatics tools (e.g., SEA, SwissTargetPrediction) to identify potential off-targets. Validate findings via CRISPR knockouts of predicted targets and functional rescue assays .
【论文必备工具】让导师对你刮目相看的SCI论文写作神器04:53
Q. Data Analysis & Validation
Q. How should researchers statistically analyze contradictory results in this compound’s dose-dependent toxicity profiles?
- Methodological Answer : Apply mixed-effects models to account for inter-study heterogeneity (e.g., differences in cell lines or dosing intervals). Use funnel plots to detect publication bias and sensitivity analyses to weigh outlier influences. Share code repositories (e.g., GitHub) for transparency .
Q. What validation strategies are critical for confirming this compound’s proposed mechanism of action in Parkinson’s disease models?
- Methodological Answer : Combine genetic knockdown (siRNA) of target receptors with behavioral assays (e.g., rotarod tests). Corrogate findings with positron emission tomography (PET) imaging of dopamine release in vivo. Require independent replication in at least two model systems before publication .
Q. Collaborative & Ethical Considerations
Q. How can interdisciplinary teams mitigate bias in this compound research?
Properties
IUPAC Name |
(6S)-6-N-[3-[[(6S)-2-amino-4,5,6,7-tetrahydro-1,3-benzothiazol-6-yl]amino]-2-methylpentyl]-4,5,6,7-tetrahydro-1,3-benzothiazole-2,6-diamine | |
---|---|---|
Source | PubChem | |
URL | https://pubchem.ncbi.nlm.nih.gov | |
Description | Data deposited in or computed by PubChem | |
InChI |
InChI=1S/C20H32N6S2/c1-3-14(24-13-5-7-16-18(9-13)28-20(22)26-16)11(2)10-23-12-4-6-15-17(8-12)27-19(21)25-15/h11-14,23-24H,3-10H2,1-2H3,(H2,21,25)(H2,22,26)/t11?,12-,13-,14?/m0/s1 | |
Source | PubChem | |
URL | https://pubchem.ncbi.nlm.nih.gov | |
Description | Data deposited in or computed by PubChem | |
InChI Key |
FDILCXYULUBXSL-QPPOZKHWSA-N | |
Source | PubChem | |
URL | https://pubchem.ncbi.nlm.nih.gov | |
Description | Data deposited in or computed by PubChem | |
Canonical SMILES |
CCC(C(C)CNC1CCC2=C(C1)SC(=N2)N)NC3CCC4=C(C3)SC(=N4)N | |
Source | PubChem | |
URL | https://pubchem.ncbi.nlm.nih.gov | |
Description | Data deposited in or computed by PubChem | |
Isomeric SMILES |
CCC(C(C)CN[C@H]1CCC2=C(C1)SC(=N2)N)N[C@H]3CCC4=C(C3)SC(=N4)N | |
Source | PubChem | |
URL | https://pubchem.ncbi.nlm.nih.gov | |
Description | Data deposited in or computed by PubChem | |
Molecular Formula |
C20H32N6S2 | |
Source | PubChem | |
URL | https://pubchem.ncbi.nlm.nih.gov | |
Description | Data deposited in or computed by PubChem | |
Molecular Weight |
420.6 g/mol | |
Source | PubChem | |
URL | https://pubchem.ncbi.nlm.nih.gov | |
Description | Data deposited in or computed by PubChem | |
Retrosynthesis Analysis
AI-Powered Synthesis Planning: Our tool employs the Template_relevance Pistachio, Template_relevance Bkms_metabolic, Template_relevance Pistachio_ringbreaker, Template_relevance Reaxys, Template_relevance Reaxys_biocatalysis model, leveraging a vast database of chemical reactions to predict feasible synthetic routes.
One-Step Synthesis Focus: Specifically designed for one-step synthesis, it provides concise and direct routes for your target compounds, streamlining the synthesis process.
Accurate Predictions: Utilizing the extensive PISTACHIO, BKMS_METABOLIC, PISTACHIO_RINGBREAKER, REAXYS, REAXYS_BIOCATALYSIS database, our tool offers high-accuracy predictions, reflecting the latest in chemical research and data.
Strategy Settings
Precursor scoring | Relevance Heuristic |
---|---|
Min. plausibility | 0.01 |
Model | Template_relevance |
Template Set | Pistachio/Bkms_metabolic/Pistachio_ringbreaker/Reaxys/Reaxys_biocatalysis |
Top-N result to add to graph | 6 |
Feasible Synthetic Routes
Disclaimer and Information on In-Vitro Research Products
Please be aware that all articles and product information presented on BenchChem are intended solely for informational purposes. The products available for purchase on BenchChem are specifically designed for in-vitro studies, which are conducted outside of living organisms. In-vitro studies, derived from the Latin term "in glass," involve experiments performed in controlled laboratory settings using cells or tissues. It is important to note that these products are not categorized as medicines or drugs, and they have not received approval from the FDA for the prevention, treatment, or cure of any medical condition, ailment, or disease. We must emphasize that any form of bodily introduction of these products into humans or animals is strictly prohibited by law. It is essential to adhere to these guidelines to ensure compliance with legal and ethical standards in research and experimentation.