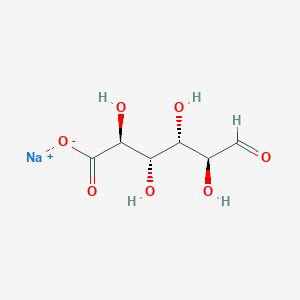
Sodium mannuronate
- Click on QUICK INQUIRY to receive a quote from our team of experts.
- With the quality product at a COMPETITIVE price, you can focus more on your research.
Overview
Description
D-Mannuronic acid (sodium) is a uronic acid monosaccharide derived from mannose. It is a component of alginic acid, a polysaccharide found predominantly in brown algae. This compound is also incorporated into some bacterial capsular polysaccharides . D-Mannuronic acid (sodium) is known for its applications in various fields, including pharmaceuticals, biotechnology, and materials science.
Mechanism of Action
Target of Action
Sodium mannuronate, a component of alginate, primarily targets the metabolic pathways of bacteria and brown algae . It is also involved in the regulation of immune responses . In the context of biomedical applications, this compound interacts with cells in the human body, particularly in the gut microbiota .
Mode of Action
This compound interacts with its targets through various mechanisms. In bacterial alginate metabolism, it is often O-acetylated at O-2 and/or O-3 of D-mannuronate, which is catalyzed by mannuronate acetylase . This acetylation can affect the ion-binding selectivity and water-binding properties of polysaccharides . In the human body, this compound may interact with immune cells and gut microbiota to exert its effects .
Biochemical Pathways
This compound is involved in the metabolic pathways of brown algae and bacteria . It is a major component of alginates, which are degraded by enzymes in these organisms . The degradation products of alginate polymers, such as alginate oligosaccharides (AOS), have shown health beneficial effects, such as immunomodulatory, antimicrobial, and antioxidant activities .
Pharmacokinetics
It is known that this compound is soluble in water , which suggests that it could be readily absorbed and distributed in the body. More research is needed to fully understand the pharmacokinetics of this compound.
Result of Action
The molecular and cellular effects of this compound’s action are diverse. In the context of alginate metabolism, it plays a crucial role in the degradation and utilization of alginates . In the human body, this compound may have various effects, such as restoring the gut microbiota and reducing helper type 1 (Th1)-related neuroinflammation in the brain .
Action Environment
Environmental factors can influence the action, efficacy, and stability of this compound. For instance, the structure of alginate derived from brown algae, which includes this compound, is influenced by factors such as season, growth environment, age, and location . Furthermore, the action of this compound in the human body may be influenced by various factors, including the individual’s diet, health status, and gut microbiota composition .
Biochemical Analysis
Biochemical Properties
Sodium mannuronate plays a significant role in biochemical reactions, particularly in the modulation of immune responses. It interacts with various enzymes and proteins, including interleukin-17 (IL-17), RAR-related orphan receptor gamma (RORγt), interleukin-4 (IL-4), and GATA binding protein 3 (GATA3). These interactions are crucial in regulating gene expressions related to inflammation and immune responses . This compound’s ability to modulate these biomolecules suggests its potential as a therapeutic agent for conditions such as rheumatoid arthritis .
Cellular Effects
This compound influences various cellular processes, including cell signaling pathways, gene expression, and cellular metabolism. It has been shown to affect the expression of genes involved in inflammatory responses, thereby modulating the activity of immune cells . Additionally, this compound can impact cellular metabolism by promoting the excretion of uric acid and modulating gut microbiota, which is particularly beneficial in conditions like hyperuricemia .
Molecular Mechanism
At the molecular level, this compound exerts its effects through binding interactions with specific biomolecules. It can inhibit or activate enzymes involved in inflammatory pathways, leading to changes in gene expression. For instance, this compound has been shown to downregulate the expression of pro-inflammatory cytokines such as IL-17 and RORγt while upregulating anti-inflammatory cytokines like IL-4 and GATA3 . These molecular interactions highlight its potential as an anti-inflammatory agent.
Temporal Effects in Laboratory Settings
In laboratory settings, the effects of this compound can vary over time. Studies have shown that its stability and degradation are influenced by factors such as temperature and storage conditions . Long-term exposure to this compound has been observed to maintain its anti-inflammatory properties, although its efficacy may diminish over extended periods due to degradation .
Dosage Effects in Animal Models
The effects of this compound in animal models are dose-dependent. At lower doses, it has been shown to exert beneficial effects such as reducing inflammation and promoting uric acid excretion . At higher doses, this compound may exhibit toxic or adverse effects, including potential impacts on liver and kidney function . These findings underscore the importance of determining optimal dosages for therapeutic applications.
Metabolic Pathways
This compound is involved in various metabolic pathways, particularly those related to the degradation of alginates. It interacts with enzymes such as alginate lyases, which break down alginate polymers into oligosaccharides . These metabolic processes are essential for the bioconversion of brown algae and have implications for the production of biofuels and other bioproducts .
Transport and Distribution
Within cells and tissues, this compound is transported and distributed through specific transporters and binding proteins. It can interact with sodium-dependent transport mechanisms, which regulate its localization and accumulation in various cellular compartments . These interactions are crucial for maintaining its bioavailability and therapeutic efficacy.
Subcellular Localization
This compound’s subcellular localization is influenced by targeting signals and post-translational modifications. It can be directed to specific compartments or organelles, such as the plasma membrane, centrosomes, and primary cilia . These localizations are essential for its activity and function, particularly in regulating cellular processes such as pH homeostasis and cell cycle progression .
Preparation Methods
Synthetic Routes and Reaction Conditions: D-Mannuronic acid (sodium) can be synthesized through the oxidation of D-mannose. The process involves the use of oxidizing agents such as nitric acid or sodium hypochlorite under controlled conditions to convert D-mannose into D-mannuronic acid. The resulting acid is then neutralized with sodium hydroxide to form the sodium salt .
Industrial Production Methods: Industrial production of D-Mannuronic acid (sodium) typically involves the extraction of alginic acid from brown algae, followed by hydrolysis to release D-mannuronic acid. The acid is then neutralized with sodium hydroxide to produce the sodium salt. This method is preferred for large-scale production due to its efficiency and cost-effectiveness .
Types of Reactions:
Oxidation: D-Mannuronic acid (sodium) can undergo oxidation reactions to form various derivatives. Common oxidizing agents include nitric acid and sodium hypochlorite.
Reduction: Reduction of D-Mannuronic acid (sodium) can yield D-mannose or other reduced forms.
Substitution: The carboxyl group in D-Mannuronic acid (sodium) can participate in substitution reactions, forming esters or amides.
Common Reagents and Conditions:
Oxidation: Nitric acid, sodium hypochlorite, and other oxidizing agents under controlled temperature and pH conditions.
Reduction: Reducing agents such as sodium borohydride or hydrogen gas in the presence of a catalyst.
Substitution: Alcohols or amines in the presence of activating agents like dicyclohexylcarbodiimide (DCC).
Major Products:
Oxidation: Various oxidized derivatives of D-Mannuronic acid.
Reduction: D-mannose and other reduced forms.
Substitution: Esters and amides of D-Mannuronic acid.
Scientific Research Applications
D-Mannuronic acid (sodium) has a wide range of applications in scientific research:
Chemistry: Used as a building block for the synthesis of complex carbohydrates and polysaccharides.
Biology: Incorporated into bacterial capsular polysaccharides, playing a role in bacterial virulence and immune evasion.
Comparison with Similar Compounds
D-Mannuronic acid (sodium) can be compared with other uronic acids such as:
L-Guluronic Acid: Another component of alginic acid, differing in its stereochemistry and biological properties.
D-Glucuronic Acid: Found in various polysaccharides and glycosaminoglycans, with distinct metabolic and structural roles.
D-Galacturonic Acid: A component of pectin, with applications in food and pharmaceutical industries.
Uniqueness: D-Mannuronic acid (sodium) is unique due to its specific incorporation into alginic acid and bacterial polysaccharides, as well as its notable anti-inflammatory and immunosuppressive properties .
Properties
CAS No. |
921-56-2 |
---|---|
Molecular Formula |
C6H10NaO7 |
Molecular Weight |
217.13 g/mol |
IUPAC Name |
sodium;(2S,3S,4S,5S)-2,3,4,5-tetrahydroxy-6-oxohexanoate |
InChI |
InChI=1S/C6H10O7.Na/c7-1-2(8)3(9)4(10)5(11)6(12)13;/h1-5,8-11H,(H,12,13);/t2-,3-,4+,5+;/m1./s1 |
InChI Key |
QKHMTHNLNZGTSP-MHFWOIHZSA-N |
SMILES |
C(=O)C(C(C(C(C(=O)[O-])O)O)O)O.[Na+] |
Isomeric SMILES |
C(=O)[C@H]([C@H]([C@@H]([C@@H](C(=O)O)O)O)O)O.[Na] |
Canonical SMILES |
C(=O)C(C(C(C(C(=O)O)O)O)O)O.[Na] |
Origin of Product |
United States |
Retrosynthesis Analysis
AI-Powered Synthesis Planning: Our tool employs the Template_relevance Pistachio, Template_relevance Bkms_metabolic, Template_relevance Pistachio_ringbreaker, Template_relevance Reaxys, Template_relevance Reaxys_biocatalysis model, leveraging a vast database of chemical reactions to predict feasible synthetic routes.
One-Step Synthesis Focus: Specifically designed for one-step synthesis, it provides concise and direct routes for your target compounds, streamlining the synthesis process.
Accurate Predictions: Utilizing the extensive PISTACHIO, BKMS_METABOLIC, PISTACHIO_RINGBREAKER, REAXYS, REAXYS_BIOCATALYSIS database, our tool offers high-accuracy predictions, reflecting the latest in chemical research and data.
Strategy Settings
Precursor scoring | Relevance Heuristic |
---|---|
Min. plausibility | 0.01 |
Model | Template_relevance |
Template Set | Pistachio/Bkms_metabolic/Pistachio_ringbreaker/Reaxys/Reaxys_biocatalysis |
Top-N result to add to graph | 6 |
Feasible Synthetic Routes
Q1: How is sodium mannuronate utilized in the development of biomaterials for bone regeneration?
A2: this compound, in conjunction with other biocompatible materials, forms the basis for innovative green hydrogels designed for oral bone defect regeneration. [] These hydrogels, incorporating this compound/guluronate, gelatin, and bioactive mineral fillers like calcium silicates and dicalcium phosphate dihydrate, offer a sustainable approach to biomaterial design. [] Studies have shown that these hydrogels exhibit desirable properties like calcium ion release, alkalizing activity, porosity, and the ability to promote apatite nucleation, all crucial for supporting bone regeneration. [] Additionally, these materials demonstrate good biocompatibility, encouraging the expression of vascular and osteogenic markers in mesenchymal stem cells. []
Q2: Can you describe a simple and efficient method for preparing this compound?
A3: A straightforward procedure for obtaining crystalline this compound starts with algin, a naturally occurring polysaccharide. [] The algin is first converted to alginic acid, which undergoes hydrolysis using concentrated and subsequently dilute sulfuric acid. [] Unlike previous methods that involved intermediate purifications, this method allows direct crystallization of this compound from the hydrolyzed alginic acid solution, resulting in a 25-30% yield. [] This simplified procedure offers a more efficient way to obtain this valuable compound.
Disclaimer and Information on In-Vitro Research Products
Please be aware that all articles and product information presented on BenchChem are intended solely for informational purposes. The products available for purchase on BenchChem are specifically designed for in-vitro studies, which are conducted outside of living organisms. In-vitro studies, derived from the Latin term "in glass," involve experiments performed in controlled laboratory settings using cells or tissues. It is important to note that these products are not categorized as medicines or drugs, and they have not received approval from the FDA for the prevention, treatment, or cure of any medical condition, ailment, or disease. We must emphasize that any form of bodily introduction of these products into humans or animals is strictly prohibited by law. It is essential to adhere to these guidelines to ensure compliance with legal and ethical standards in research and experimentation.