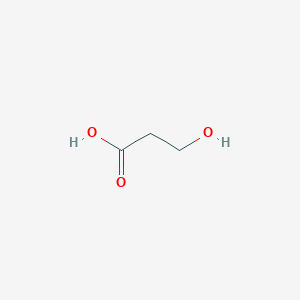
3-Hydroxypropionic acid
Overview
Description
3-Hydroxypropionic acid (3-HP) is a β-hydroxy acid that is soluble in water and many oxygenated organic solvents . It is a 3-carbon carboxylic acid, structural isomer of lactic acid, which naturally exists in some thermophilic archaea and bacteria . It is an acidic viscous liquid with a pKa of 4.9 .
Synthesis Analysis
3-HP can be obtained by base-induced hydration of acrylic acid followed by reacidification . Another synthesis involves cyanation of ethylene chlorohydrin followed by hydrolysis of the resulting nitrile . Hydrolysis of propiolactone is yet another route . Bioproduction of 3-HP has attracted more attention due to utilization of renewable biomass .Chemical Reactions Analysis
3-HP represents an economically important platform compound from which a panel of bulk chemicals can be derived . Upon distillation, it dehydrates to form acrylic acid .Physical And Chemical Properties Analysis
3-HP is an acidic viscous liquid with a pKa of 4.9 . It is very soluble in water, soluble in ethanol and diethyl ether .Scientific Research Applications
Precursor for Chemical Synthesis
3-Hydroxypropionic acid (3-HP) serves as an important platform chemical used as a precursor in the production of added-value compounds such as acrylic acid . It is utilized in industrial processes for synthesizing various chemicals, particularly acrylates .
Biodegradable Plastics
One of the significant applications of 3-HP is its polymerization to yield poly(3-hydroxypropionate) (P-3HP) , a type of biodegradable plastic. This application is particularly notable due to its environmental benefits and the sustainable properties of the resulting material .
Cosmetics and Pharmaceuticals
3-HP finds applications in the formulation of products within the cosmetics and pharmaceutical industries. Its inclusion in these products is due to its chemical properties that can enhance product performance and stability .
Bio-based Acrylic Acid
The microbial synthesis of 3-HP is a green and sustainable method that has gained attention for producing bio-based acrylic acid. This approach leverages renewable resources and biotechnological advancements .
Microbial Fermentation
Microbial fermentation processes have been developed to produce 3-HP using engineered yeast, Escherichia coli, cyanobacteria, and other microorganisms. This method emphasizes the use of renewable substrates like glycerol and glucose .
Genetic Engineering Research
Studies involving genetic engineering have focused on increasing the expression of heterologous genes in microorganisms like Methylorubrum extorquens to achieve high levels of 3-HP production. This research provides valuable insights into genetic manipulation techniques .
Mechanism of Action
Target of Action
3-Hydroxypropionic acid (3-HP) is a valuable platform compound from which a range of bulk chemicals can be derived . The primary targets of 3-HP are the key enzymes involved in its biosynthesis pathways, such as glycerol dehydratase (PduCDE) and propionaldehyde dehydrogenase (PduP) . These enzymes play a crucial role in the conversion of glycerol to 3-HP .
Safety and Hazards
Future Directions
3-HP is considered as a potential building block either for organic synthesis or high performance polymers . It has attracted significant attention in recent years due to its green and sustainable properties . Future research may focus on improving the production yield of 3-HP from renewable resources .
properties
IUPAC Name |
3-hydroxypropanoic acid | |
---|---|---|
Source | PubChem | |
URL | https://pubchem.ncbi.nlm.nih.gov | |
Description | Data deposited in or computed by PubChem | |
InChI |
InChI=1S/C3H6O3/c4-2-1-3(5)6/h4H,1-2H2,(H,5,6) | |
Source | PubChem | |
URL | https://pubchem.ncbi.nlm.nih.gov | |
Description | Data deposited in or computed by PubChem | |
InChI Key |
ALRHLSYJTWAHJZ-UHFFFAOYSA-N | |
Source | PubChem | |
URL | https://pubchem.ncbi.nlm.nih.gov | |
Description | Data deposited in or computed by PubChem | |
Canonical SMILES |
C(CO)C(=O)O | |
Source | PubChem | |
URL | https://pubchem.ncbi.nlm.nih.gov | |
Description | Data deposited in or computed by PubChem | |
Molecular Formula |
C3H6O3 | |
Source | PubChem | |
URL | https://pubchem.ncbi.nlm.nih.gov | |
Description | Data deposited in or computed by PubChem | |
Related CAS |
25718-95-0 | |
Record name | Poly(3-hydroxypropionate) | |
Source | CAS Common Chemistry | |
URL | https://commonchemistry.cas.org/detail?cas_rn=25718-95-0 | |
Description | CAS Common Chemistry is an open community resource for accessing chemical information. Nearly 500,000 chemical substances from CAS REGISTRY cover areas of community interest, including common and frequently regulated chemicals, and those relevant to high school and undergraduate chemistry classes. This chemical information, curated by our expert scientists, is provided in alignment with our mission as a division of the American Chemical Society. | |
Explanation | The data from CAS Common Chemistry is provided under a CC-BY-NC 4.0 license, unless otherwise stated. | |
DSSTOX Substance ID |
DTXSID50198305 | |
Record name | Hydracrylic acid | |
Source | EPA DSSTox | |
URL | https://comptox.epa.gov/dashboard/DTXSID50198305 | |
Description | DSSTox provides a high quality public chemistry resource for supporting improved predictive toxicology. | |
Molecular Weight |
90.08 g/mol | |
Source | PubChem | |
URL | https://pubchem.ncbi.nlm.nih.gov | |
Description | Data deposited in or computed by PubChem | |
Physical Description |
Solid | |
Record name | Hydroxypropionic acid | |
Source | Human Metabolome Database (HMDB) | |
URL | http://www.hmdb.ca/metabolites/HMDB0000700 | |
Description | The Human Metabolome Database (HMDB) is a freely available electronic database containing detailed information about small molecule metabolites found in the human body. | |
Explanation | HMDB is offered to the public as a freely available resource. Use and re-distribution of the data, in whole or in part, for commercial purposes requires explicit permission of the authors and explicit acknowledgment of the source material (HMDB) and the original publication (see the HMDB citing page). We ask that users who download significant portions of the database cite the HMDB paper in any resulting publications. | |
Product Name |
3-Hydroxypropionic acid | |
CAS RN |
503-66-2 | |
Record name | 3-Hydroxypropionic acid | |
Source | CAS Common Chemistry | |
URL | https://commonchemistry.cas.org/detail?cas_rn=503-66-2 | |
Description | CAS Common Chemistry is an open community resource for accessing chemical information. Nearly 500,000 chemical substances from CAS REGISTRY cover areas of community interest, including common and frequently regulated chemicals, and those relevant to high school and undergraduate chemistry classes. This chemical information, curated by our expert scientists, is provided in alignment with our mission as a division of the American Chemical Society. | |
Explanation | The data from CAS Common Chemistry is provided under a CC-BY-NC 4.0 license, unless otherwise stated. | |
Record name | Hydracrylic acid | |
Source | ChemIDplus | |
URL | https://pubchem.ncbi.nlm.nih.gov/substance/?source=chemidplus&sourceid=0000503662 | |
Description | ChemIDplus is a free, web search system that provides access to the structure and nomenclature authority files used for the identification of chemical substances cited in National Library of Medicine (NLM) databases, including the TOXNET system. | |
Record name | Hydracrylic acid | |
Source | DrugBank | |
URL | https://www.drugbank.ca/drugs/DB03688 | |
Description | The DrugBank database is a unique bioinformatics and cheminformatics resource that combines detailed drug (i.e. chemical, pharmacological and pharmaceutical) data with comprehensive drug target (i.e. sequence, structure, and pathway) information. | |
Explanation | Creative Common's Attribution-NonCommercial 4.0 International License (http://creativecommons.org/licenses/by-nc/4.0/legalcode) | |
Record name | Hydracrylic acid | |
Source | EPA DSSTox | |
URL | https://comptox.epa.gov/dashboard/DTXSID50198305 | |
Description | DSSTox provides a high quality public chemistry resource for supporting improved predictive toxicology. | |
Record name | 3-hydroxypropionic acid | |
Source | European Chemicals Agency (ECHA) | |
URL | https://echa.europa.eu/substance-information/-/substanceinfo/100.007.250 | |
Description | The European Chemicals Agency (ECHA) is an agency of the European Union which is the driving force among regulatory authorities in implementing the EU's groundbreaking chemicals legislation for the benefit of human health and the environment as well as for innovation and competitiveness. | |
Explanation | Use of the information, documents and data from the ECHA website is subject to the terms and conditions of this Legal Notice, and subject to other binding limitations provided for under applicable law, the information, documents and data made available on the ECHA website may be reproduced, distributed and/or used, totally or in part, for non-commercial purposes provided that ECHA is acknowledged as the source: "Source: European Chemicals Agency, http://echa.europa.eu/". Such acknowledgement must be included in each copy of the material. ECHA permits and encourages organisations and individuals to create links to the ECHA website under the following cumulative conditions: Links can only be made to webpages that provide a link to the Legal Notice page. | |
Record name | HYDRACRYLIC ACID | |
Source | FDA Global Substance Registration System (GSRS) | |
URL | https://gsrs.ncats.nih.gov/ginas/app/beta/substances/C4ZF6XLD2X | |
Description | The FDA Global Substance Registration System (GSRS) enables the efficient and accurate exchange of information on what substances are in regulated products. Instead of relying on names, which vary across regulatory domains, countries, and regions, the GSRS knowledge base makes it possible for substances to be defined by standardized, scientific descriptions. | |
Explanation | Unless otherwise noted, the contents of the FDA website (www.fda.gov), both text and graphics, are not copyrighted. They are in the public domain and may be republished, reprinted and otherwise used freely by anyone without the need to obtain permission from FDA. Credit to the U.S. Food and Drug Administration as the source is appreciated but not required. | |
Record name | Hydroxypropionic acid | |
Source | Human Metabolome Database (HMDB) | |
URL | http://www.hmdb.ca/metabolites/HMDB0000700 | |
Description | The Human Metabolome Database (HMDB) is a freely available electronic database containing detailed information about small molecule metabolites found in the human body. | |
Explanation | HMDB is offered to the public as a freely available resource. Use and re-distribution of the data, in whole or in part, for commercial purposes requires explicit permission of the authors and explicit acknowledgment of the source material (HMDB) and the original publication (see the HMDB citing page). We ask that users who download significant portions of the database cite the HMDB paper in any resulting publications. | |
Melting Point |
< 25 °C | |
Record name | Hydroxypropionic acid | |
Source | Human Metabolome Database (HMDB) | |
URL | http://www.hmdb.ca/metabolites/HMDB0000700 | |
Description | The Human Metabolome Database (HMDB) is a freely available electronic database containing detailed information about small molecule metabolites found in the human body. | |
Explanation | HMDB is offered to the public as a freely available resource. Use and re-distribution of the data, in whole or in part, for commercial purposes requires explicit permission of the authors and explicit acknowledgment of the source material (HMDB) and the original publication (see the HMDB citing page). We ask that users who download significant portions of the database cite the HMDB paper in any resulting publications. | |
Synthesis routes and methods
Procedure details
Retrosynthesis Analysis
AI-Powered Synthesis Planning: Our tool employs the Template_relevance Pistachio, Template_relevance Bkms_metabolic, Template_relevance Pistachio_ringbreaker, Template_relevance Reaxys, Template_relevance Reaxys_biocatalysis model, leveraging a vast database of chemical reactions to predict feasible synthetic routes.
One-Step Synthesis Focus: Specifically designed for one-step synthesis, it provides concise and direct routes for your target compounds, streamlining the synthesis process.
Accurate Predictions: Utilizing the extensive PISTACHIO, BKMS_METABOLIC, PISTACHIO_RINGBREAKER, REAXYS, REAXYS_BIOCATALYSIS database, our tool offers high-accuracy predictions, reflecting the latest in chemical research and data.
Strategy Settings
Precursor scoring | Relevance Heuristic |
---|---|
Min. plausibility | 0.01 |
Model | Template_relevance |
Template Set | Pistachio/Bkms_metabolic/Pistachio_ringbreaker/Reaxys/Reaxys_biocatalysis |
Top-N result to add to graph | 6 |
Feasible Synthetic Routes
Q & A
Q1: What makes 3-hydroxypropionic acid (3-HP) an attractive platform chemical?
A1: 3-HP holds significant potential as a platform chemical due to its versatility in synthesizing various valuable compounds. It serves as a key precursor for producing biodegradable polymers like poly(3-hydroxypropionate) (P-3HP), offering a sustainable alternative to conventional plastics []. Additionally, 3-HP finds applications in synthesizing acrylic acid and its derivatives, widely used in products such as diapers, plastics, and paints [].
Q2: Can microorganisms be used to produce 3-HP?
A2: Yes, microbial production of 3-HP has gained considerable attention as a green and sustainable alternative to traditional chemical synthesis. Researchers are exploring various metabolic pathways and engineering microorganisms to enhance 3-HP production efficiency []. For instance, recombinant Escherichia coli strains have been developed to produce 3-HP from glycerol, a readily available and renewable feedstock [, , ].
Q3: What are the challenges associated with microbial 3-HP production?
A3: One challenge is the toxicity of 3-HP to the microorganisms themselves. High concentrations of 3-HP can inhibit cell growth and hinder production efficiency. Researchers are investigating strategies to enhance 3-HP tolerance in microorganisms. For instance, adaptive laboratory evolution has been successfully applied to generate Saccharomyces cerevisiae strains with significantly improved tolerance to 3-HP []. Another challenge is optimizing fermentation processes and downstream separation methods to improve the overall economic viability of microbial 3-HP production.
Q4: How can wheat and sugar beet byproducts contribute to 3-HP production?
A4: Research has explored utilizing agricultural coproducts like wheat and sugar beet residues as cost-effective growth media for Lactobacillus reuteri DSM17938, a bacterium capable of converting glycerol to 3-HP. Optimizing the media composition using response surface methodology led to increased 3-HP production, showcasing the potential of these coproducts for sustainable bioproduction [].
Q5: Can 3-HP be co-produced with other valuable chemicals?
A6: Yes, co-production of 3-HP with other valuable chemicals like 1,3-propanediol (1,3-PDO) from glycerol has been proposed as a strategy to improve the economic feasibility of bioprocesses. Studies have explored utilizing Klebsiella pneumoniae strains for the co-production of 3-HP and 1,3-PDO under different oxygen conditions [, ].
Q6: Which metabolic pathways are involved in 3-HP production?
A6: Several metabolic pathways can lead to 3-HP production in microorganisms. These include:
- Glycerol pathway: Glycerol is dehydrated to 3-hydroxypropionaldehyde, subsequently oxidized to 3-HP [, , , ].
- Malonyl-CoA pathway: Malonyl-CoA is reduced to malonic semialdehyde and further reduced to 3-HP [, , ].
- β-alanine pathway: β-alanine is converted to 3-HP via a series of enzymatic reactions [].
Q7: How is 3-HP production regulated in microorganisms?
A7: The regulation of 3-HP biosynthesis is complex and involves various factors, including:
- Enzyme levels: The expression and activity of enzymes involved in 3-HP pathways are crucial for efficient production [].
- Cofactor availability: Availability of cofactors like coenzyme B12 and NADPH is essential for optimal enzyme function [, ].
- Metabolic flux: Directing metabolic flux towards 3-HP production by engineering specific pathways is critical [, ].
Q8: Can genetic engineering improve 3-HP production?
A8: Yes, genetic engineering strategies are widely employed to enhance 3-HP production in microorganisms:
- Overexpressing key enzymes: Increasing the expression of enzymes involved in 3-HP pathways can boost production [, , , , ].
- Introducing heterologous pathways: Introducing genes from other organisms can establish new or enhance existing 3-HP pathways [, , ].
- Modifying regulatory mechanisms: Altering regulatory mechanisms can redirect metabolic flux towards 3-HP production [, ].
Q9: How can the accumulation of toxic intermediates be mitigated during 3-HP production?
A10: One example involves controlling glycerol addition during fermentation with Lactobacillus reuteri. This approach effectively reduced the accumulation of the toxic intermediate 3-hydroxypropionaldehyde (3-HPA), contributing to a higher final 3-HP concentration and improved biocatalyst performance [].
Q10: Does 3-HP itself regulate its biosynthesis?
A11: Research has identified a 3-HP inducible promoter system in Paracoccus denitrificans. This system utilizes a LysR-type transcriptional regulator and a specific DNA sequence to control gene expression in response to 3-HP levels. This finding presents an opportunity to develop biosensors and optimize 3-HP production by fine-tuning gene expression based on real-time 3-HP concentrations [].
Q11: What is the molecular formula and weight of 3-hydroxypropionic acid?
A11: The molecular formula of 3-hydroxypropionic acid is C3H6O3, and its molecular weight is 90.08 g/mol.
Q12: What are some methods for analyzing 3-HP?
A13: High-performance liquid chromatography (HPLC) is commonly used to determine 3-HP concentration in fermentation broths and other samples [, , ]. Gas chromatography coupled with mass spectrometry (GC-MS) enables the identification and quantification of 3-HP and its metabolites in various matrices [, , ].
Q13: How can 3-HP be separated and purified from fermentation broths?
A13: Various downstream processing methods can be employed for 3-HP recovery and purification, including:
- Reactive extraction: This technique utilizes extractants like tri-n-octylamine and Aliquat 336 in n-decanol to selectively separate 3-HP from aqueous solutions [].
- Electrodialysis: This method separates and concentrates 3-HP salts from aqueous solutions by applying an electric current across ion-exchange membranes [].
- Crystallization: Purified 3-HP can be obtained through crystallization processes such as suspension or layer crystallization [].
Q14: What are potential applications of 3-HP beyond biodegradable plastics?
A14: Beyond its use in biodegradable plastics, 3-HP holds promise for various applications, including:
- Synthesis of other chemicals: 3-HP can be further converted into valuable chemicals like acrylic acid, 1,3-propanediol, and malonic acid [, ].
- Food and feed additives: 3-HP has potential applications as a food preservative and animal feed supplement [].
- Pharmaceutical intermediates: 3-HP can be used as a building block for synthesizing pharmaceuticals and drug delivery systems [, ].
Q15: What are the future directions for 3-HP research?
A15: Future research on 3-HP will likely focus on:
- Developing more efficient and robust microbial cell factories for 3-HP production. This includes exploring novel metabolic pathways, engineering more tolerant strains, and optimizing fermentation processes [, ].
- Improving downstream processing technologies for cost-effective 3-HP recovery and purification. This involves optimizing existing methods and exploring new techniques like membrane separation and chromatography [, , ].
- Expanding the range of applications for 3-HP and its derivatives. This includes exploring its use in new bio-based materials, pharmaceuticals, and other value-added products [, , ].
Disclaimer and Information on In-Vitro Research Products
Please be aware that all articles and product information presented on BenchChem are intended solely for informational purposes. The products available for purchase on BenchChem are specifically designed for in-vitro studies, which are conducted outside of living organisms. In-vitro studies, derived from the Latin term "in glass," involve experiments performed in controlled laboratory settings using cells or tissues. It is important to note that these products are not categorized as medicines or drugs, and they have not received approval from the FDA for the prevention, treatment, or cure of any medical condition, ailment, or disease. We must emphasize that any form of bodily introduction of these products into humans or animals is strictly prohibited by law. It is essential to adhere to these guidelines to ensure compliance with legal and ethical standards in research and experimentation.