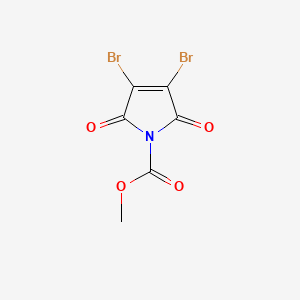
Methyl 3,4-dibromo-2,5-dioxo-2,5-dihydro-1H-pyrrole-1-carboxylate
Overview
Description
Methyl 3,4-dibromo-2,5-dioxo-2,5-dihydro-1H-pyrrole-1-carboxylate (CAS: 1442447-48-4) is a brominated pyrrole derivative with the molecular formula C₆H₃Br₂NO₄ and a molecular weight of 312.90 g/mol . Structurally, it features a pyrrole ring substituted with two bromine atoms at positions 3 and 4, two ketone groups at positions 2 and 5, and a methyl ester at position 1. This compound is typically stored at 2–8°C under dry conditions, indicating sensitivity to thermal degradation or hydrolysis .
Biochemical Analysis
Biochemical Properties
Methyl 3,4-dibromo-2,5-dioxo-2,5-dihydro-1H-pyrrole-1-carboxylate plays a significant role in biochemical reactions due to its ability to act as a covalent ligand for thiol groups. This compound can label cysteine residues in proteins, which is crucial for studying protein structure and function. The presence of two leaving groups allows it to bind two thiols simultaneously, facilitating the formation of larger molecular structures . Additionally, the methyl ester group can be saponified to link the compound to amines, further expanding its utility in biochemical applications .
Cellular Effects
This compound has been observed to influence various cellular processes. It can affect cell signaling pathways, gene expression, and cellular metabolism. By labeling cysteine residues in proteins, this compound can alter protein function and interactions, leading to changes in cellular behavior. For instance, it may impact the activity of enzymes involved in critical metabolic pathways, thereby influencing overall cellular metabolism .
Molecular Mechanism
The molecular mechanism of action of this compound involves its interaction with thiol groups in proteins. The unsaturated dibromo motif acts as a covalent ligand, forming stable bonds with cysteine residues. This interaction can lead to enzyme inhibition or activation, depending on the specific protein and context. Additionally, the compound’s ability to bind two thiols simultaneously allows it to crosslink proteins, potentially affecting their structure and function .
Temporal Effects in Laboratory Settings
In laboratory settings, the effects of this compound can change over time. The compound’s stability and degradation are critical factors that influence its long-term effects on cellular function. Studies have shown that the compound remains stable under specific conditions, but its activity may decrease over time due to degradation . Long-term exposure to the compound in in vitro or in vivo studies can lead to sustained changes in cellular behavior, highlighting the importance of understanding its temporal effects .
Dosage Effects in Animal Models
The effects of this compound vary with different dosages in animal models. At lower doses, the compound may exhibit beneficial effects, such as modulating enzyme activity or influencing metabolic pathways. At higher doses, it can cause toxic or adverse effects, including cellular damage or disruption of normal cellular processes . Understanding the dosage effects is crucial for determining the compound’s therapeutic potential and safety profile.
Metabolic Pathways
This compound is involved in various metabolic pathways. It interacts with enzymes and cofactors that play essential roles in cellular metabolism. By labeling cysteine residues, the compound can affect the activity of enzymes involved in critical metabolic processes, leading to changes in metabolic flux and metabolite levels . These interactions highlight the compound’s potential to modulate metabolic pathways and influence overall cellular function.
Transport and Distribution
The transport and distribution of this compound within cells and tissues are influenced by its interactions with transporters and binding proteins. The compound’s ability to label cysteine residues allows it to be transported and localized to specific cellular compartments. This localization can affect its accumulation and activity within cells, influencing its overall biochemical effects .
Subcellular Localization
This compound exhibits specific subcellular localization patterns. The compound’s targeting signals and post-translational modifications direct it to particular compartments or organelles within the cell. This localization is crucial for its activity and function, as it allows the compound to interact with specific biomolecules and exert its biochemical effects .
Biological Activity
Methyl 3,4-dibromo-2,5-dioxo-2,5-dihydro-1H-pyrrole-1-carboxylate (CAS No. 1442447-48-4) is a synthetic compound with notable biological activities. This article provides a comprehensive overview of its biochemical properties, cellular effects, and molecular mechanisms, supported by data tables and relevant research findings.
Molecular Formula and Weight:
- Molecular Formula: CHBrNO
- Molecular Weight: 312.90 g/mol
Physical Properties:
Property | Value |
---|---|
CAS Number | 1442447-48-4 |
Boiling Point | Not available |
Storage Conditions | Sealed in dry, 2-8°C |
This compound acts primarily as a covalent ligand for thiol groups in proteins. This ability allows it to label cysteine residues, which is crucial for studying protein structure and function. The compound's structure includes two leaving groups that enable it to bind two thiols simultaneously, facilitating the formation of larger molecular structures and potentially altering protein interactions.
Cellular Effects
Research indicates that this compound influences various cellular processes:
- Cell Signaling Pathways: It can modulate signal transduction pathways by altering the activity of proteins involved in these pathways.
- Gene Expression: By affecting transcription factors and other regulatory proteins, it can influence gene expression patterns.
- Cellular Metabolism: The compound impacts enzymes involved in metabolic pathways, thereby influencing overall cellular metabolism.
The primary mechanism involves the interaction with thiol groups in proteins through its unsaturated dibromo motif. This interaction can lead to either enzyme inhibition or activation depending on the specific context:
- Covalent Bond Formation: The compound forms stable bonds with cysteine residues.
- Crosslinking Proteins: Its ability to bind two thiols allows for crosslinking, which may alter protein structure and function.
Temporal Effects in Laboratory Settings
The stability and degradation of this compound are critical for its long-term effects on cellular functions. Studies have shown that while the compound remains stable under specific conditions, its biological activity may diminish over time due to degradation processes.
Case Studies and Research Findings
Several studies have highlighted the biological activity of this compound:
- Antimicrobial Activity: In vitro tests demonstrated that pyrrole derivatives exhibit significant antibacterial activity against both Gram-positive and Gram-negative bacteria. For instance, related compounds showed minimum inhibitory concentration (MIC) values ranging from 0.0039 to 0.025 mg/mL against Staphylococcus aureus and Escherichia coli .
- Protein Interaction Studies: Research has shown that the compound effectively labels cysteine residues in various proteins, allowing for detailed studies on protein dynamics and interactions .
Scientific Research Applications
Biochemical Research
Covalent Labeling of Proteins
One of the primary applications of this compound is its ability to act as a covalent ligand for thiol groups in proteins. This property enables it to label cysteine residues, which is crucial for studying protein structure and function. The compound can bind two thiols simultaneously due to its dibromo motif, facilitating the formation of larger molecular structures and potentially altering protein interactions and activities.
Impact on Cellular Processes
Research indicates that methyl 3,4-dibromo-2,5-dioxo-2,5-dihydro-1H-pyrrole-1-carboxylate can influence various cellular processes. By modifying proteins through cysteine labeling, it can affect cell signaling pathways and gene expression. This interaction may lead to changes in enzyme activity within critical metabolic pathways, thereby influencing overall cellular metabolism .
Synthetic Chemistry
Synthesis of Complex Molecules
The compound serves as an important intermediate in the synthesis of various organic molecules. Its unique structure allows chemists to use it as a building block for creating more complex compounds. For instance, it has been utilized in the synthesis of pyrroline nitroxides and other derivatives that have potential applications in materials science and medicinal chemistry .
Reactivity with Other Compounds
this compound exhibits reactivity that can be harnessed in organic synthesis. Its ability to form stable bonds with nucleophiles makes it a valuable reagent for developing new chemical entities .
Case Studies and Research Findings
Q & A
Basic Research Questions
Q. What are the standard synthetic routes for Methyl 3,4-dibromo-2,5-dioxo-2,5-dihydro-1H-pyrrole-1-carboxylate, and how can reaction conditions be optimized?
The compound is typically synthesized via bromination of a pyrrole-dione precursor. For example, dibromination at the 3,4-positions can be achieved using bromine or N-bromosuccinimide (NBS) in a halogenated solvent (e.g., dichloromethane) under controlled temperature (0–25°C). Optimization involves monitoring reaction progress via TLC or NMR to avoid over-bromination. Post-synthesis, purification is performed using column chromatography (silica gel, ethyl acetate/hexane eluent) or recrystallization from ethanol .
Q. How can spectroscopic techniques (NMR, IR) be used to confirm the structure of this compound?
- 1H/13C NMR : The ester carbonyl (C=O) appears at ~165–170 ppm (13C). The pyrrole-dione ring carbons (C2/C5) resonate near 160–170 ppm (13C), while brominated carbons (C3/C4) show deshielded signals (~110–120 ppm). Methoxy groups typically appear as singlets at ~3.8–4.0 ppm (1H) .
- IR : Strong absorption bands at ~1750 cm⁻¹ (ester C=O) and ~1700 cm⁻¹ (pyrrole-dione C=O) confirm functional groups. Bromine substituents may cause minor shifts in fingerprint regions .
Q. What crystallographic methods are recommended for resolving its molecular structure?
Single-crystal X-ray diffraction (SCXRD) is ideal. Crystals are grown via slow evaporation of a saturated solution in dichloromethane/hexane. Data collection at 293 K using Mo-Kα radiation (λ = 0.71073 Å) and refinement via SHELXL-97 (for small molecules) or SHELXTL (for disorder handling) ensures accurate bond lengths/angles. Hydrogen bonding and packing are analyzed using PLATON or Mercury .
Advanced Research Questions
Q. How can crystallographic disorder in derivatives of this compound be resolved during refinement?
Ethoxy or alkyl side chains often exhibit positional disorder. In such cases:
- Use PART commands in SHELXL to model split positions.
- Apply constraints (e.g., DFIX, SIMU) to maintain reasonable geometry.
- Validate with R1 convergence (<5%) and check residual electron density maps (<0.5 eÅ⁻³) .
Q. What computational methods (DFT, MD) are suitable for studying its electronic properties and reactivity?
- DFT : Optimize geometry at B3LYP/6-31G(d) level to calculate frontier molecular orbitals (HOMO/LUMO), electrostatic potential (ESP), and Fukui indices for electrophilic/nucleophilic sites.
- Molecular Dynamics (MD) : Simulate solvation effects in toluene or DMSO using OPLS-AA force fields to predict solubility and aggregation behavior .
Q. How can conflicting NMR data (e.g., unexpected coupling patterns) be analyzed for derivatives?
- Dynamic Effects : Use variable-temperature NMR (VT-NMR) to detect conformational exchange (e.g., hindered rotation of substituents).
- 2D NMR : Employ COSY and HSQC to resolve overlapping signals. For example, coupling between pyrrole protons and adjacent bromine atoms may cause splitting anomalies .
Q. What strategies mitigate side reactions during functionalization (e.g., Suzuki coupling)?
- Protection of reactive sites : Temporarily protect the pyrrole-dione carbonyls as acetals to prevent undesired nucleophilic attack.
- Catalyst optimization : Use Pd(PPh3)4 with K2CO3 in THF/H2O (3:1) at 80°C. Monitor via LC-MS to detect cross-coupling by-products .
Q. How does hydrogen bonding influence crystal packing, and how is this analyzed?
Graph set analysis (as per Etter’s rules) identifies recurring hydrogen-bond motifs (e.g., R22(10) rings). Tools like CrystalExplorer generate Hirshfeld surfaces to quantify intermolecular interactions (e.g., Br···O contacts). For example, C–H···O bonds often stabilize layered packing parallel to the c-axis .
Application-Oriented Questions
Q. What role does this compound play in designing bioactive molecules (e.g., kinase inhibitors)?
The electron-deficient pyrrole-dione core serves as a Michael acceptor for nucleophilic residues (e.g., cysteine thiols in kinases). Bromine atoms enhance electrophilicity and provide sites for further functionalization (e.g., cross-coupling to attach pharmacophores) .
Q. How can its stability under varying pH conditions be assessed for drug formulation studies?
- pH-rate profiling : Incubate the compound in buffers (pH 1–12) at 37°C. Monitor degradation via HPLC-UV at 254 nm.
- LC-MS : Identify hydrolysis products (e.g., decarboxylation or ester cleavage) .
Q. Data Validation & Reproducibility
Q. What metrics ensure reliable crystallographic data deposition?
- CheckCIF/PLATON : Validate symmetry, ADP mismatches, and twinning.
- CSD guidelines : Report R1/wR2 values, completeness (>95%), and Flack parameter for chiral centers .
Q. How can batch-to-batch variability in synthesis be minimized?
- DoE (Design of Experiments) : Use response surface methodology (RSM) to optimize bromination time/temperature.
- QC protocols : Standardize NMR (≥95% purity), HPLC (retention time matching), and elemental analysis (C, H, N within ±0.4%) .
Comparison with Similar Compounds
Table 1: Structural and Physicochemical Comparison
Note: Molecular weight for 1-(2,4-Dichlorophenyl)-1H-pyrrole-2,5-dione is estimated based on formula.
Key Comparative Analysis
The methyl ester group at position 1 may influence solubility in organic solvents compared to ethyl esters (e.g., the compound in ) or amides .
Applications: Fluoroimide (C₁₀H₄Cl₂FNO₂) is explicitly used as a pesticide, leveraging its halogenated structure for bioactivity . The target compound’s lack of direct application data implies it may serve as a synthetic precursor for brominated agrochemicals or pharmaceuticals .
Synthesis and Stability :
- Brominated pyrroles like the target compound often require controlled bromination conditions (e.g., using Br₂ or NBS) to avoid overhalogenation .
- Chlorinated analogs (e.g., fluoroimide) may be synthesized via Friedel-Crafts alkylation or Ullmann coupling, reflecting differences in halogen reactivity .
Crystallography and Hydrogen Bonding :
- The diketone motif in the target compound could engage in C=O···H hydrogen bonding , influencing crystal packing. This contrasts with amide-containing analogs (e.g., ’s compound), which form stronger N–H···O bonds .
- Software like SHELX and WinGX () are critical for resolving such structural nuances via single-crystal X-ray diffraction .
Properties
IUPAC Name |
methyl 3,4-dibromo-2,5-dioxopyrrole-1-carboxylate | |
---|---|---|
Source | PubChem | |
URL | https://pubchem.ncbi.nlm.nih.gov | |
Description | Data deposited in or computed by PubChem | |
InChI |
InChI=1S/C6H3Br2NO4/c1-13-6(12)9-4(10)2(7)3(8)5(9)11/h1H3 | |
Source | PubChem | |
URL | https://pubchem.ncbi.nlm.nih.gov | |
Description | Data deposited in or computed by PubChem | |
InChI Key |
YVESAOJZLFCAHI-UHFFFAOYSA-N | |
Source | PubChem | |
URL | https://pubchem.ncbi.nlm.nih.gov | |
Description | Data deposited in or computed by PubChem | |
Canonical SMILES |
COC(=O)N1C(=O)C(=C(C1=O)Br)Br | |
Source | PubChem | |
URL | https://pubchem.ncbi.nlm.nih.gov | |
Description | Data deposited in or computed by PubChem | |
Molecular Formula |
C6H3Br2NO4 | |
Source | PubChem | |
URL | https://pubchem.ncbi.nlm.nih.gov | |
Description | Data deposited in or computed by PubChem | |
Molecular Weight |
312.90 g/mol | |
Source | PubChem | |
URL | https://pubchem.ncbi.nlm.nih.gov | |
Description | Data deposited in or computed by PubChem | |
Retrosynthesis Analysis
AI-Powered Synthesis Planning: Our tool employs the Template_relevance Pistachio, Template_relevance Bkms_metabolic, Template_relevance Pistachio_ringbreaker, Template_relevance Reaxys, Template_relevance Reaxys_biocatalysis model, leveraging a vast database of chemical reactions to predict feasible synthetic routes.
One-Step Synthesis Focus: Specifically designed for one-step synthesis, it provides concise and direct routes for your target compounds, streamlining the synthesis process.
Accurate Predictions: Utilizing the extensive PISTACHIO, BKMS_METABOLIC, PISTACHIO_RINGBREAKER, REAXYS, REAXYS_BIOCATALYSIS database, our tool offers high-accuracy predictions, reflecting the latest in chemical research and data.
Strategy Settings
Precursor scoring | Relevance Heuristic |
---|---|
Min. plausibility | 0.01 |
Model | Template_relevance |
Template Set | Pistachio/Bkms_metabolic/Pistachio_ringbreaker/Reaxys/Reaxys_biocatalysis |
Top-N result to add to graph | 6 |
Feasible Synthetic Routes
Disclaimer and Information on In-Vitro Research Products
Please be aware that all articles and product information presented on BenchChem are intended solely for informational purposes. The products available for purchase on BenchChem are specifically designed for in-vitro studies, which are conducted outside of living organisms. In-vitro studies, derived from the Latin term "in glass," involve experiments performed in controlled laboratory settings using cells or tissues. It is important to note that these products are not categorized as medicines or drugs, and they have not received approval from the FDA for the prevention, treatment, or cure of any medical condition, ailment, or disease. We must emphasize that any form of bodily introduction of these products into humans or animals is strictly prohibited by law. It is essential to adhere to these guidelines to ensure compliance with legal and ethical standards in research and experimentation.