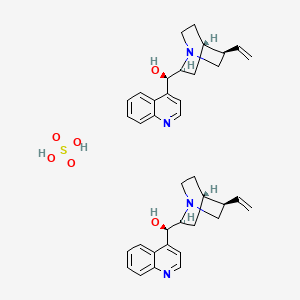
Cinchonidine sulphate
Overview
Description
Cinchonidine sulfate is a naturally occurring alkaloid derived from the bark of Cinchona trees. It is one of the four major Cinchona alkaloids, alongside quinine, quinidine, and cinchonine . Historically, Cinchona alkaloids have been significant due to their antimalarial properties, with quinine being the most well-known . Cinchonidine sulfate is primarily used for its medicinal properties and in various chemical applications.
Mechanism of Action
Target of Action
Cinchonidine sulphate, a major member of the Cinchona alkaloids, is known for its significant impact on human civilization .
Mode of Action
It is generally assumed that cinchona alkaloids, including this compound, prevent the polymerization of the toxic hematin (aquafe(iii)protoporphyrin ix) formed by the degradation of hemoglobin in erythrocytes to hemozoin (β-hematin) .
Biochemical Pathways
This compound affects the biochemical pathway involved in the degradation of hemoglobin in erythrocytes. By preventing the polymerization of toxic hematin to hemozoin, it disrupts the life cycle of the malaria vector Plasmodium falciparum .
Pharmacokinetics
It has been reported that the mean plasma concentration for cinchonidine is approximately 25 mg/L .
Result of Action
The molecular and cellular effects of this compound’s action primarily involve the disruption of the life cycle of the malaria vector Plasmodium falciparum. By preventing the polymerization of toxic hematin to hemozoin, it inhibits the growth and multiplication of the malaria parasite .
Biochemical Analysis
Biochemical Properties
Cinchonidine sulphate is known for its role in biochemical reactions, particularly in its interaction with enzymes and proteins. It interacts with cytochrome P450 enzymes, which are involved in the metabolism of various drugs. The compound also exhibits inhibitory effects on certain enzymes, such as acetylcholinesterase, which is crucial for neurotransmission. These interactions highlight the importance of this compound in modulating biochemical pathways and influencing physiological processes .
Cellular Effects
This compound has been shown to exert protective effects on endothelial cells. It attenuates indoxyl sulphate-induced cell damage by downregulating the p53 signaling pathway and promoting MDM2 cytoplasmic-nuclear shuttling. This results in reduced cell death, cellular senescence, and improved vasculogenic activity in human umbilical vein endothelial cells (HUVECs). Additionally, this compound influences cell signaling pathways, gene expression, and cellular metabolism, making it a potential therapeutic agent for endothelial dysfunction .
Molecular Mechanism
At the molecular level, this compound exerts its effects through various mechanisms. It binds to specific biomolecules, leading to enzyme inhibition or activation. For instance, it inhibits acetylcholinesterase, thereby affecting neurotransmission. The compound also modulates gene expression by influencing transcription factors and signaling pathways. These molecular interactions are crucial for understanding the therapeutic potential of this compound .
Temporal Effects in Laboratory Settings
In laboratory settings, the effects of this compound have been observed to change over time. The compound’s stability and degradation are critical factors that influence its long-term effects on cellular function. Studies have shown that this compound remains stable under specific conditions, but its efficacy may decrease over time due to degradation. Long-term exposure to this compound has been associated with sustained protective effects on endothelial cells, highlighting its potential for chronic therapeutic use .
Dosage Effects in Animal Models
The effects of this compound vary with different dosages in animal models. At low doses, the compound exhibits therapeutic effects, such as reducing muscle cramps and improving endothelial function. At high doses, this compound may cause toxic or adverse effects, including gastrointestinal disturbances and neurotoxicity. These dosage-dependent effects are crucial for determining the safe and effective use of this compound in therapeutic applications .
Metabolic Pathways
This compound is involved in various metabolic pathways, primarily mediated by cytochrome P450 enzymes. These enzymes catalyze the hydroxylation and glucuronidation of this compound, leading to its elimination from the body. The compound’s metabolism also affects metabolic flux and metabolite levels, influencing its pharmacokinetics and pharmacodynamics .
Transport and Distribution
Within cells and tissues, this compound is transported and distributed through specific transporters and binding proteins. These interactions determine the compound’s localization and accumulation in different cellular compartments. The transport and distribution of this compound are essential for its therapeutic efficacy and safety .
Subcellular Localization
This compound exhibits specific subcellular localization, which affects its activity and function. The compound is directed to particular compartments or organelles through targeting signals and post-translational modifications. This subcellular localization is crucial for understanding the precise mechanisms of action of this compound and its potential therapeutic applications .
Preparation Methods
Synthetic Routes and Reaction Conditions
Cinchonidine sulfate is typically extracted from the bark of Cinchona trees. The extraction process involves several steps:
Harvesting and Drying: The bark is harvested and dried.
Extraction: The dried bark is treated with an acidic solution to extract the alkaloids.
Purification: The crude extract is purified using various chemical processes, including crystallization and filtration.
Industrial Production Methods
In industrial settings, the extraction process is scaled up to handle large quantities of bark. The hot and clear acidic liquor is neutralized with a hot sodium hydroxide solution at a pH of 5.5 and then cooled. Upon cooling, crude quinine sulfate crystallizes out and is centrifuged. It is further refined by boiling with water and activated carbon to obtain pure quinine sulfate . Similar methods are used for the extraction and purification of cinchonidine sulfate.
Chemical Reactions Analysis
Types of Reactions
Cinchonidine sulfate undergoes various chemical reactions, including:
Oxidation: It can be oxidized to form different derivatives.
Reduction: Reduction reactions can modify its structure.
Substitution: It can undergo substitution reactions to form new compounds.
Common Reagents and Conditions
Oxidation: Common oxidizing agents include potassium permanganate and hydrogen peroxide.
Reduction: Reducing agents such as sodium borohydride are used.
Substitution: Various halogenating agents and nucleophiles are employed.
Major Products
The major products formed from these reactions include various derivatives of cinchonidine, which can be used in further chemical synthesis and applications .
Scientific Research Applications
Cinchonidine sulfate has a wide range of applications in scientific research:
Comparison with Similar Compounds
Similar Compounds
Quinine: Known for its antimalarial properties.
Quinidine: Used as an antiarrhythmic agent.
Cinchonine: Another Cinchona alkaloid with similar properties.
Uniqueness
Cinchonidine sulfate is unique due to its specific stereochemistry, which makes it valuable in asymmetric synthesis. Its ability to act as a chiral catalyst sets it apart from other Cinchona alkaloids .
Properties
CAS No. |
524-61-8 |
---|---|
Molecular Formula |
C19H24N2O5S |
Molecular Weight |
392.5 g/mol |
IUPAC Name |
(R)-[(2S,4S,5R)-5-ethenyl-1-azabicyclo[2.2.2]octan-2-yl]-quinolin-4-ylmethanol;sulfuric acid |
InChI |
InChI=1S/C19H22N2O.H2O4S/c1-2-13-12-21-10-8-14(13)11-18(21)19(22)16-7-9-20-17-6-4-3-5-15(16)17;1-5(2,3)4/h2-7,9,13-14,18-19,22H,1,8,10-12H2;(H2,1,2,3,4)/t13-,14-,18-,19+;/m0./s1 |
InChI Key |
KFHZMXDENPHHOU-YYXOUSRLSA-N |
SMILES |
C=CC1CN2CCC1CC2C(C3=CC=NC4=CC=CC=C34)O.C=CC1CN2CCC1CC2C(C3=CC=NC4=CC=CC=C34)O.OS(=O)(=O)O |
Isomeric SMILES |
C=C[C@H]1CN2CC[C@H]1C[C@H]2[C@@H](C3=CC=NC4=CC=CC=C34)O.OS(=O)(=O)O |
Canonical SMILES |
C=CC1CN2CCC1CC2C(C3=CC=NC4=CC=CC=C34)O.OS(=O)(=O)O |
Pictograms |
Irritant |
Origin of Product |
United States |
Retrosynthesis Analysis
AI-Powered Synthesis Planning: Our tool employs the Template_relevance Pistachio, Template_relevance Bkms_metabolic, Template_relevance Pistachio_ringbreaker, Template_relevance Reaxys, Template_relevance Reaxys_biocatalysis model, leveraging a vast database of chemical reactions to predict feasible synthetic routes.
One-Step Synthesis Focus: Specifically designed for one-step synthesis, it provides concise and direct routes for your target compounds, streamlining the synthesis process.
Accurate Predictions: Utilizing the extensive PISTACHIO, BKMS_METABOLIC, PISTACHIO_RINGBREAKER, REAXYS, REAXYS_BIOCATALYSIS database, our tool offers high-accuracy predictions, reflecting the latest in chemical research and data.
Strategy Settings
Precursor scoring | Relevance Heuristic |
---|---|
Min. plausibility | 0.01 |
Model | Template_relevance |
Template Set | Pistachio/Bkms_metabolic/Pistachio_ringbreaker/Reaxys/Reaxys_biocatalysis |
Top-N result to add to graph | 6 |
Feasible Synthetic Routes
Q1: What adverse effects have been observed with the topical use of cinchonidine sulphate?
A1: A case study [] reported a 26-year-old male experiencing a vesicular dermatitis with intense itching on his scalp, face, and neck after using a hair tonic containing this compound. The reaction occurred within 24 hours of application and was characterized by tense, acuminate vesicles filled with clear to straw-colored fluid. The patient also experienced edema of the scalp and eyes, facial swelling, nausea, abdominal distress, and loose stools. Patch testing confirmed the this compound-containing ingredient as the cause of the reaction.
Q2: Has this compound been explored for any applications beyond its known antimalarial properties?
A2: Interestingly, a historical study [] investigated the use of this compound in reducing the prevalence of "fever" cases in jails. While the exact methodology and results are not detailed in the abstract, this highlights an attempt to leverage the potential antipyretic properties of this compound in a specific setting.
Q3: Are there any documented historical reviews or critiques of research involving this compound?
A3: While not directly focusing on this compound itself, a book review [] mentioning the compound provides a glimpse into the broader scientific discourse of that time. This suggests the existence of historical scientific literature potentially containing valuable insights into the early research and applications of this compound.
Disclaimer and Information on In-Vitro Research Products
Please be aware that all articles and product information presented on BenchChem are intended solely for informational purposes. The products available for purchase on BenchChem are specifically designed for in-vitro studies, which are conducted outside of living organisms. In-vitro studies, derived from the Latin term "in glass," involve experiments performed in controlled laboratory settings using cells or tissues. It is important to note that these products are not categorized as medicines or drugs, and they have not received approval from the FDA for the prevention, treatment, or cure of any medical condition, ailment, or disease. We must emphasize that any form of bodily introduction of these products into humans or animals is strictly prohibited by law. It is essential to adhere to these guidelines to ensure compliance with legal and ethical standards in research and experimentation.