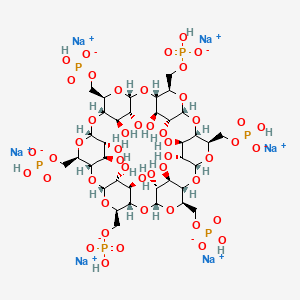
alpha-Cyclodextrin phosphate sodium salt
Overview
Description
Alpha-Cyclodextrin phosphate sodium salt is a derivative of alpha-cyclodextrin, a cyclic oligosaccharide composed of six glucose units linked by alpha-1,4-glycosidic bonds. This compound is known for its ability to form inclusion complexes with various guest molecules, enhancing their solubility and stability. The phosphate groups attached to the cyclodextrin ring further modify its chemical properties, making it useful in a variety of scientific and industrial applications .
Mechanism of Action
Target of Action
Alpha-Cyclodextrin phosphate sodium salt primarily targets lipids and has been shown to alter plasma lipid levels . It forms a hydrophobic central cavity that binds lipids . This compound is also known to interact with various small and large drug molecules, building aqueous soluble inclusion complexes .
Mode of Action
The interaction of this compound with its targets involves the formation of inclusion complexes. The compound’s cyclical structure, composed of glucose subunits, forms a hydrophobic central cavity that can encapsulate lipophilic compounds . This encapsulation improves the stability and water solubility of the guest molecules .
Biochemical Pathways
this compound affects several biochemical pathways. It inhibits carbohydrate, fat, and cholesterol absorption by encapsulating digestive enzymes, phospholipids, and food ingredients . It also has various health-promoting effects exerted via effects on the gut microbiota, which include anti-obesity and anti-atherosclerotic effects, the amelioration of constipation, bone strengthening, the improvement of gut immunity, the amelioration of allergic disease, and the improvement of exercise performance .
Pharmacokinetics
The pharmacokinetics of this compound are influenced by its ability to form inclusion complexes with various compounds. This property enhances the solubility of the compound, especially in water and in ethanol:water mixtures . This improved solubility is accompanied by enhanced solubilizing effect, which is beneficial for the bioavailability of the compound .
Result of Action
The action of this compound results in a variety of effects at the molecular and cellular level. It has been observed to cause a modest reduction in small LDL particles and an improvement in glucose-related parameters . Furthermore, it has been shown to have various health-promoting effects, including anti-obesity and anti-atherosclerotic effects, the amelioration of constipation, bone strengthening, the improvement of gut immunity, the amelioration of allergic disease, and the improvement of exercise performance .
Action Environment
The action, efficacy, and stability of this compound are influenced by various environmental factors. Furthermore, its prebiotic effects differ from those of conventional beneficial fermentable dietary fibers, as it affects Lactobacillus and Bacteroides, which readily generate short-chain fatty acids .
Biochemical Analysis
Biochemical Properties
Alpha-Cyclodextrin phosphate sodium salt plays a significant role in biochemical reactions due to its ability to form inclusion complexes with various biomolecules. This compound interacts with enzymes, proteins, and other biomolecules through its hydrophobic cavity, which can encapsulate guest molecules. For instance, this compound can bind to lipids, enhancing their solubility and stability . Additionally, it can interact with digestive enzymes, such as amylase, by forming complexes that inhibit enzyme activity . This interaction is crucial in modulating the bioavailability of certain nutrients and drugs.
Cellular Effects
This compound influences various cellular processes by altering the solubility and availability of biomolecules. It has been shown to affect cell signaling pathways, gene expression, and cellular metabolism. For example, this compound can reduce the solubility of lipids in the small intestine, thereby inhibiting their absorption . This effect can lead to changes in lipid metabolism and a reduction in serum cholesterol levels. Furthermore, this compound can modulate the gut microbiota, promoting the growth of beneficial bacteria and improving gut health .
Molecular Mechanism
The molecular mechanism of this compound involves its ability to form inclusion complexes with various biomolecules. This compound can encapsulate lipids, phospholipids, and other hydrophobic molecules within its hydrophobic cavity . The binding interactions are primarily driven by van der Waals forces and hydrophobic interactions. Additionally, this compound can inhibit the activity of digestive enzymes by forming complexes that block the active sites of these enzymes . This inhibition can lead to changes in nutrient absorption and metabolism.
Temporal Effects in Laboratory Settings
In laboratory settings, the effects of this compound can change over time due to its stability and degradation properties. This compound is relatively stable under physiological conditions, but it can undergo hydrolysis and degradation over extended periods . Long-term studies have shown that this compound can maintain its biochemical activity for several weeks, but its efficacy may decrease over time due to gradual degradation . Additionally, the long-term effects on cellular function have been observed in both in vitro and in vivo studies, indicating potential benefits in reducing lipid absorption and improving metabolic health .
Dosage Effects in Animal Models
The effects of this compound vary with different dosages in animal models. At low doses, this compound has been shown to reduce lipid absorption and improve metabolic parameters without causing significant adverse effects . At higher doses, this compound can lead to gastrointestinal discomfort and other adverse effects . Threshold effects have been observed, where the beneficial effects plateau at certain dosages, and further increases in dosage do not result in additional benefits .
Metabolic Pathways
This compound is involved in various metabolic pathways, particularly those related to lipid metabolism. This compound can inhibit the absorption of dietary lipids by forming complexes with digestive enzymes and phospholipids . Additionally, this compound can modulate the gut microbiota, promoting the production of short-chain fatty acids that have beneficial effects on metabolic health . The interactions with enzymes and cofactors involved in lipid metabolism are crucial for its effects on metabolic flux and metabolite levels .
Transport and Distribution
The transport and distribution of this compound within cells and tissues are influenced by its interactions with transporters and binding proteins. This compound can be transported across cell membranes through passive diffusion and facilitated transport mechanisms . Once inside the cells, this compound can accumulate in specific tissues, such as the liver and intestines, where it exerts its biochemical effects . The distribution within tissues is also influenced by its ability to form complexes with various biomolecules .
Subcellular Localization
This compound exhibits specific subcellular localization patterns that are crucial for its activity and function. This compound can be localized to the cytoplasm, where it interacts with various enzymes and proteins involved in metabolic processes . Additionally, this compound can be targeted to specific organelles, such as the endoplasmic reticulum and lysosomes, through post-translational modifications and targeting signals . These localization patterns are essential for its role in modulating cellular metabolism and function.
Preparation Methods
Synthetic Routes and Reaction Conditions
The synthesis of alpha-Cyclodextrin phosphate sodium salt typically involves the phosphorylation of alpha-cyclodextrin. This can be achieved by reacting alpha-cyclodextrin with phosphorylating agents such as phosphorus pentoxide or phosphorus oxychloride in the presence of an amide-based solvent . The reaction conditions, including temperature and reaction time, are carefully controlled to ensure the efficient introduction of phosphate groups onto the cyclodextrin ring.
Industrial Production Methods
In industrial settings, the production of this compound follows similar synthetic routes but on a larger scale. The process involves the use of large reactors and precise control of reaction parameters to achieve high yields and purity. The final product is typically purified through crystallization or other separation techniques to remove any unreacted starting materials and by-products .
Chemical Reactions Analysis
Types of Reactions
Alpha-Cyclodextrin phosphate sodium salt can undergo various chemical reactions, including:
Oxidation: The compound can be oxidized under specific conditions to introduce additional functional groups.
Reduction: Reduction reactions can modify the phosphate groups or other substituents on the cyclodextrin ring.
Substitution: The phosphate groups can be substituted with other functional groups through nucleophilic substitution reactions.
Common Reagents and Conditions
Common reagents used in these reactions include oxidizing agents like hydrogen peroxide, reducing agents such as sodium borohydride, and nucleophiles like amines or thiols. The reaction conditions, including solvent choice, temperature, and pH, are optimized based on the desired transformation .
Major Products
The major products formed from these reactions depend on the specific reagents and conditions used. For example, oxidation may yield cyclodextrin derivatives with additional hydroxyl or carbonyl groups, while substitution reactions can introduce a wide range of functional groups onto the cyclodextrin ring .
Scientific Research Applications
Alpha-Cyclodextrin phosphate sodium salt has a wide range of applications in scientific research, including:
Comparison with Similar Compounds
Similar Compounds
Beta-Cyclodextrin phosphate sodium salt: Composed of seven glucose units, it has a larger cavity size compared to alpha-Cyclodextrin phosphate sodium salt.
Gamma-Cyclodextrin phosphate sodium salt: Contains eight glucose units, offering an even larger cavity for inclusion complexes.
Uniqueness
This compound is unique due to its smaller cavity size, which makes it suitable for encapsulating smaller guest molecules. Additionally, the presence of phosphate groups enhances its solubility and stability, making it particularly useful in aqueous environments .
Properties
IUPAC Name |
hexasodium;[(1S,3R,5R,6S,8R,10R,11S,13R,15R,16S,18R,20R,21S,23R,25R,26S,28R,30R,31R,32R,33R,34R,35R,36R,37R,38R,39R,40R,41R,42R)-31,32,33,34,35,36,37,38,39,40,41,42-dodecahydroxy-10,15,20,25,30-pentakis[[hydroxy(oxido)phosphoryl]oxymethyl]-2,4,7,9,12,14,17,19,22,24,27,29-dodecaoxaheptacyclo[26.2.2.23,6.28,11.213,16.218,21.223,26]dotetracontan-5-yl]methyl hydrogen phosphate | |
---|---|---|
Source | PubChem | |
URL | https://pubchem.ncbi.nlm.nih.gov | |
Description | Data deposited in or computed by PubChem | |
InChI |
InChI=1S/C36H66O48P6.6Na/c37-13-19(43)31-73-7(1-67-85(49,50)51)25(13)79-32-20(44)14(38)27(9(74-32)3-69-87(55,56)57)81-34-22(46)16(40)29(11(76-34)5-71-89(61,62)63)83-36-24(48)18(42)30(12(78-36)6-72-90(64,65)66)84-35-23(47)17(41)28(10(77-35)4-70-88(58,59)60)82-33-21(45)15(39)26(80-31)8(75-33)2-68-86(52,53)54;;;;;;/h7-48H,1-6H2,(H2,49,50,51)(H2,52,53,54)(H2,55,56,57)(H2,58,59,60)(H2,61,62,63)(H2,64,65,66);;;;;;/q;6*+1/p-6/t7-,8-,9-,10-,11-,12-,13-,14-,15-,16-,17-,18-,19-,20-,21-,22-,23-,24-,25-,26-,27-,28-,29-,30-,31-,32-,33-,34-,35-,36-;;;;;;/m1....../s1 | |
Source | PubChem | |
URL | https://pubchem.ncbi.nlm.nih.gov | |
Description | Data deposited in or computed by PubChem | |
InChI Key |
UVXCHNWIGVNOSW-WWKXUIADSA-H | |
Source | PubChem | |
URL | https://pubchem.ncbi.nlm.nih.gov | |
Description | Data deposited in or computed by PubChem | |
Canonical SMILES |
C(C1C2C(C(C(O1)OC3C(OC(C(C3O)O)OC4C(OC(C(C4O)O)OC5C(OC(C(C5O)O)OC6C(OC(C(C6O)O)OC7C(OC(O2)C(C7O)O)COP(=O)(O)[O-])COP(=O)(O)[O-])COP(=O)(O)[O-])COP(=O)(O)[O-])COP(=O)(O)[O-])O)O)OP(=O)(O)[O-].[Na+].[Na+].[Na+].[Na+].[Na+].[Na+] | |
Source | PubChem | |
URL | https://pubchem.ncbi.nlm.nih.gov | |
Description | Data deposited in or computed by PubChem | |
Isomeric SMILES |
C([C@@H]1[C@@H]2[C@@H]([C@H]([C@H](O1)O[C@@H]3[C@H](O[C@@H]([C@@H]([C@H]3O)O)O[C@@H]4[C@H](O[C@@H]([C@@H]([C@H]4O)O)O[C@@H]5[C@H](O[C@@H]([C@@H]([C@H]5O)O)O[C@@H]6[C@H](O[C@@H]([C@@H]([C@H]6O)O)O[C@@H]7[C@H](O[C@H](O2)[C@@H]([C@H]7O)O)COP(=O)(O)[O-])COP(=O)(O)[O-])COP(=O)(O)[O-])COP(=O)(O)[O-])COP(=O)(O)[O-])O)O)OP(=O)(O)[O-].[Na+].[Na+].[Na+].[Na+].[Na+].[Na+] | |
Source | PubChem | |
URL | https://pubchem.ncbi.nlm.nih.gov | |
Description | Data deposited in or computed by PubChem | |
Molecular Formula |
C36H60Na6O48P6 | |
Source | PubChem | |
URL | https://pubchem.ncbi.nlm.nih.gov | |
Description | Data deposited in or computed by PubChem | |
DSSTOX Substance ID |
DTXSID80746752 | |
Record name | PUBCHEM_71312276 | |
Source | EPA DSSTox | |
URL | https://comptox.epa.gov/dashboard/DTXSID80746752 | |
Description | DSSTox provides a high quality public chemistry resource for supporting improved predictive toxicology. | |
Molecular Weight |
1584.6 g/mol | |
Source | PubChem | |
URL | https://pubchem.ncbi.nlm.nih.gov | |
Description | Data deposited in or computed by PubChem | |
CAS No. |
199684-60-1 | |
Record name | PUBCHEM_71312276 | |
Source | EPA DSSTox | |
URL | https://comptox.epa.gov/dashboard/DTXSID80746752 | |
Description | DSSTox provides a high quality public chemistry resource for supporting improved predictive toxicology. | |
Retrosynthesis Analysis
AI-Powered Synthesis Planning: Our tool employs the Template_relevance Pistachio, Template_relevance Bkms_metabolic, Template_relevance Pistachio_ringbreaker, Template_relevance Reaxys, Template_relevance Reaxys_biocatalysis model, leveraging a vast database of chemical reactions to predict feasible synthetic routes.
One-Step Synthesis Focus: Specifically designed for one-step synthesis, it provides concise and direct routes for your target compounds, streamlining the synthesis process.
Accurate Predictions: Utilizing the extensive PISTACHIO, BKMS_METABOLIC, PISTACHIO_RINGBREAKER, REAXYS, REAXYS_BIOCATALYSIS database, our tool offers high-accuracy predictions, reflecting the latest in chemical research and data.
Strategy Settings
Precursor scoring | Relevance Heuristic |
---|---|
Min. plausibility | 0.01 |
Model | Template_relevance |
Template Set | Pistachio/Bkms_metabolic/Pistachio_ringbreaker/Reaxys/Reaxys_biocatalysis |
Top-N result to add to graph | 6 |
Feasible Synthetic Routes
Disclaimer and Information on In-Vitro Research Products
Please be aware that all articles and product information presented on BenchChem are intended solely for informational purposes. The products available for purchase on BenchChem are specifically designed for in-vitro studies, which are conducted outside of living organisms. In-vitro studies, derived from the Latin term "in glass," involve experiments performed in controlled laboratory settings using cells or tissues. It is important to note that these products are not categorized as medicines or drugs, and they have not received approval from the FDA for the prevention, treatment, or cure of any medical condition, ailment, or disease. We must emphasize that any form of bodily introduction of these products into humans or animals is strictly prohibited by law. It is essential to adhere to these guidelines to ensure compliance with legal and ethical standards in research and experimentation.