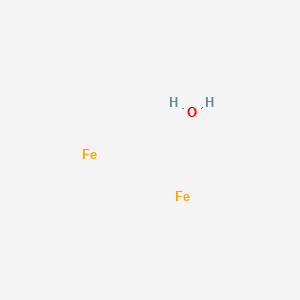
iron;hydrate
- Click on QUICK INQUIRY to receive a quote from our team of experts.
- With the quality product at a COMPETITIVE price, you can focus more on your research.
Overview
Description
Iron hydrate, commonly referred to as hydrated iron oxide, is a compound that consists of iron, oxygen, and water molecules. The most familiar form of iron hydrate is rust, which is scientifically known as hydrated iron (III) oxide. This compound forms when iron reacts with water and oxygen, resulting in a reddish-brown flaky substance. Iron hydrates are significant in various fields due to their unique chemical properties and widespread occurrence.
Preparation Methods
Synthetic Routes and Reaction Conditions
Iron hydrate can be synthesized through several methods. One common laboratory method involves the reaction of iron salts with water and oxygen. For example, iron (III) chloride can be dissolved in water, and upon exposure to air, it forms hydrated iron (III) oxide. The reaction can be represented as: [ \text{4FeCl}_3 + 3\text{O}_2 + 6\text{H}_2\text{O} \rightarrow 4\text{Fe(OH)}_3 + 12\text{HCl} ]
Industrial Production Methods
Industrially, iron hydrate is often produced as a byproduct of steel manufacturing. When iron or steel is exposed to moist air, it undergoes oxidation, forming rust. This process is accelerated in the presence of saltwater or acidic conditions. Additionally, iron hydrate can be produced through the controlled oxidation of iron in water treatment plants to remove impurities.
Chemical Reactions Analysis
Types of Reactions
Iron hydrate primarily undergoes oxidation and reduction reactions. The most common reaction is the oxidation of iron to form hydrated iron (III) oxide: [ \text{4Fe} + 3\text{O}_2 + 6\text{H}_2\text{O} \rightarrow 4\text{Fe(OH)}_3 ]
Common Reagents and Conditions
The formation of iron hydrate typically requires the presence of water and oxygen. In laboratory settings, reagents such as iron (III) chloride or iron (II) sulfate can be used. The reaction conditions often involve exposure to air or the addition of oxidizing agents.
Major Products Formed
The primary product formed from the oxidation of iron is hydrated iron (III) oxide, commonly known as rust. This compound can further dehydrate to form iron (III) oxide.
Scientific Research Applications
Iron hydrate has numerous applications in scientific research and industry:
Chemistry: Iron hydrate is used as a catalyst in various chemical reactions, including the synthesis of organic compounds.
Biology: In biological research, iron hydrate nanoparticles are used for targeted drug delivery and imaging due to their magnetic properties.
Medicine: Iron hydrate is utilized in the treatment of iron deficiency anemia. It is also used in the development of contrast agents for magnetic resonance imaging (MRI).
Industry: Iron hydrate is employed in water treatment processes to remove contaminants. It is also used as a pigment in paints and coatings.
Mechanism of Action
The mechanism by which iron hydrate exerts its effects depends on its application. In the context of catalysis, iron hydrate provides active sites for chemical reactions, facilitating the conversion of reactants to products. In biological applications, iron hydrate nanoparticles interact with cellular components, enabling targeted drug delivery and imaging. The magnetic properties of iron hydrate play a crucial role in these processes.
Comparison with Similar Compounds
Iron hydrate can be compared with other iron compounds such as iron (II) oxide and iron (III) oxide. While iron (II) oxide is a black powder and iron (III) oxide is a red-brown powder, iron hydrate is characterized by its flaky, reddish-brown appearance. Unlike its anhydrous counterparts, iron hydrate contains water molecules, which influence its chemical behavior and applications.
Similar Compounds
Iron (II) oxide (FeO): A black powder used in ceramics and as a pigment.
Iron (III) oxide (Fe2O3): A red-brown powder used in pigments and as a polishing agent.
Iron (III) chloride (FeCl3): A yellow-brown compound used in water treatment and as a catalyst.
Iron hydrate’s unique properties, such as its ability to form in the presence of water and oxygen, distinguish it from these similar compounds.
Properties
CAS No. |
61674-70-2 |
---|---|
Molecular Formula |
Fe2H2O |
Molecular Weight |
129.71 g/mol |
IUPAC Name |
iron;hydrate |
InChI |
InChI=1S/2Fe.H2O/h;;1H2 |
InChI Key |
IQGILBZPWWYYSR-UHFFFAOYSA-N |
Canonical SMILES |
O.[Fe].[Fe] |
Origin of Product |
United States |
Disclaimer and Information on In-Vitro Research Products
Please be aware that all articles and product information presented on BenchChem are intended solely for informational purposes. The products available for purchase on BenchChem are specifically designed for in-vitro studies, which are conducted outside of living organisms. In-vitro studies, derived from the Latin term "in glass," involve experiments performed in controlled laboratory settings using cells or tissues. It is important to note that these products are not categorized as medicines or drugs, and they have not received approval from the FDA for the prevention, treatment, or cure of any medical condition, ailment, or disease. We must emphasize that any form of bodily introduction of these products into humans or animals is strictly prohibited by law. It is essential to adhere to these guidelines to ensure compliance with legal and ethical standards in research and experimentation.