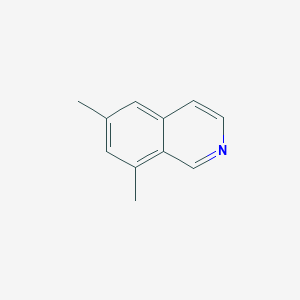
6,8-Dimethylisoquinoline
Overview
Description
6,8-Dimethylisoquinoline is an organic compound with the molecular formula C₁₁H₁₁N. It belongs to the class of isoquinolines, which are aromatic polycyclic compounds containing a benzene ring fused to a pyridine ring. This compound is characterized by the presence of two methyl groups at the 6th and 8th positions of the isoquinoline structure .
Mechanism of Action
Target of Action
This compound is a derivative of isoquinoline, a heterocyclic aromatic organic compound
Mode of Action
Isoquinolines and their derivatives are known to interact with various biological targets, potentially leading to changes in cellular processes
Biochemical Pathways
Isoquinolines and their derivatives can influence various biochemical pathways, depending on their specific structures and targets
Pharmacokinetics
These properties are crucial for understanding the bioavailability of a compound
Result of Action
The effects of a compound at the molecular and cellular levels depend on its specific targets and mode of action
Action Environment
Factors such as temperature, pH, and the presence of other compounds can influence the action of a compound
Biochemical Analysis
Biochemical Properties
6,8-Dimethylisoquinoline plays a significant role in various biochemical reactions. It interacts with several enzymes, proteins, and other biomolecules, influencing their activity and function. For instance, this compound has been shown to interact with cytochrome P450 enzymes, which are crucial for the metabolism of many drugs and endogenous compounds . The nature of these interactions often involves the binding of this compound to the active site of the enzyme, leading to either inhibition or modulation of enzymatic activity. Additionally, this compound can form complexes with metal ions, further affecting its biochemical behavior and interactions with other biomolecules .
Cellular Effects
The effects of this compound on various types of cells and cellular processes are profound. This compound has been observed to influence cell signaling pathways, gene expression, and cellular metabolism. For example, this compound can modulate the activity of signaling pathways such as the MAPK/ERK pathway, which is involved in cell proliferation and differentiation . Furthermore, it can alter gene expression by interacting with transcription factors and other regulatory proteins, leading to changes in the expression levels of specific genes. These effects on cellular metabolism and signaling pathways highlight the potential of this compound as a tool for studying cellular processes and developing therapeutic strategies.
Molecular Mechanism
The molecular mechanism of action of this compound involves several key interactions at the molecular level. This compound can bind to specific biomolecules, such as enzymes and receptors, leading to changes in their activity and function. For instance, this compound has been shown to inhibit the activity of certain kinases by binding to their active sites, thereby preventing substrate phosphorylation . Additionally, it can modulate gene expression by interacting with DNA and transcription factors, leading to changes in the transcriptional activity of target genes. These molecular interactions underline the versatility of this compound in influencing various biochemical and cellular processes.
Temporal Effects in Laboratory Settings
In laboratory settings, the effects of this compound can change over time due to factors such as stability, degradation, and long-term impact on cellular function. Studies have shown that this compound is relatively stable under standard laboratory conditions, but its stability can be affected by factors such as temperature and pH . Over time, degradation products of this compound may form, potentially altering its biological activity and effects on cells. Long-term exposure to this compound in in vitro and in vivo studies has revealed sustained effects on cellular function, including changes in cell viability, proliferation, and differentiation .
Dosage Effects in Animal Models
The effects of this compound vary with different dosages in animal models. At lower doses, this compound may exhibit beneficial effects, such as anti-inflammatory and antioxidant properties . At higher doses, it can induce toxic or adverse effects, including hepatotoxicity and nephrotoxicity . These threshold effects highlight the importance of determining the optimal dosage for therapeutic applications while minimizing potential side effects. Animal studies have provided valuable insights into the dose-dependent effects of this compound, guiding its potential use in clinical settings.
Metabolic Pathways
This compound is involved in several metabolic pathways, interacting with various enzymes and cofactors. One of the key metabolic pathways includes its oxidation by cytochrome P450 enzymes, leading to the formation of hydroxylated metabolites . These metabolites can further undergo conjugation reactions, such as glucuronidation and sulfation, facilitating their excretion from the body. The involvement of this compound in these metabolic pathways underscores its potential impact on metabolic flux and metabolite levels, influencing overall cellular metabolism.
Transport and Distribution
The transport and distribution of this compound within cells and tissues are mediated by specific transporters and binding proteins. For example, this compound can be transported across cell membranes by organic cation transporters, facilitating its uptake into cells . Once inside the cell, it can bind to intracellular proteins, influencing its localization and accumulation in specific cellular compartments. The distribution of this compound within tissues is also influenced by factors such as blood flow and tissue permeability, affecting its overall bioavailability and therapeutic potential.
Subcellular Localization
The subcellular localization of this compound plays a crucial role in its activity and function. This compound can be targeted to specific cellular compartments, such as the nucleus, mitochondria, or endoplasmic reticulum, depending on its interactions with targeting signals and post-translational modifications . For instance, this compound may be directed to the nucleus by nuclear localization signals, where it can interact with DNA and transcription factors to modulate gene expression. The subcellular localization of this compound is essential for understanding its precise mechanisms of action and potential therapeutic applications.
Preparation Methods
Synthetic Routes and Reaction Conditions
One of the synthetic routes for 6,8-Dimethylisoquinoline involves the methoximation of 2,4-dimethoxyacetophenone, followed by a methoxime-directed ruthenium-catalyzed allylation . This method employs CeCl₃·7H₂O as a promoter for the methoximation step. The reaction conditions typically include the use of a ruthenium catalyst and an appropriate solvent under controlled temperature and pressure.
Industrial Production Methods
Industrial production methods for this compound are not extensively documented in the literature. the principles of large-scale organic synthesis, such as optimization of reaction conditions, use of cost-effective reagents, and implementation of efficient purification techniques, would be applied to scale up the laboratory synthesis methods.
Chemical Reactions Analysis
Types of Reactions
6,8-Dimethylisoquinoline can undergo various types of chemical reactions, including:
Oxidation: This compound can be oxidized to form corresponding quinoline derivatives.
Reduction: Reduction reactions can convert it into dihydroisoquinoline derivatives.
Substitution: Electrophilic and nucleophilic substitution reactions can introduce different functional groups at various positions on the isoquinoline ring.
Common Reagents and Conditions
Oxidation: Common oxidizing agents include potassium permanganate (KMnO₄) and chromium trioxide (CrO₃).
Reduction: Reducing agents such as lithium aluminum hydride (LiAlH₄) and sodium borohydride (NaBH₄) are typically used.
Substitution: Reagents like halogens (e.g., bromine, chlorine) and nucleophiles (e.g., amines, thiols) are employed under appropriate conditions.
Major Products Formed
The major products formed from these reactions depend on the specific reagents and conditions used. For example, oxidation may yield quinoline derivatives, while reduction can produce dihydroisoquinoline compounds. Substitution reactions can introduce various functional groups, leading to a wide range of isoquinoline derivatives.
Scientific Research Applications
6,8-Dimethylisoquinoline has several applications in scientific research, including:
Chemistry: It is used as a building block in the synthesis of more complex organic molecules.
Biology: The compound is studied for its potential biological activities, including antimicrobial and anticancer properties.
Medicine: Research is ongoing to explore its potential as a therapeutic agent.
Industry: It is used in the development of materials with specific properties, such as dyes and polymers.
Comparison with Similar Compounds
Similar Compounds
1,3-Dimethylisoquinoline-6,8-diol: This compound has hydroxy groups at positions 6 and 8, in addition to methyl groups at positions 1 and 3.
Isoquinolinol: This compound contains a hydroxyl group on the isoquinoline ring.
Uniqueness
6,8-Dimethylisoquinoline is unique due to its specific substitution pattern, which imparts distinct chemical and biological properties. The presence of methyl groups at the 6th and 8th positions influences its reactivity and interaction with other molecules, making it a valuable compound for various applications in research and industry.
Properties
IUPAC Name |
6,8-dimethylisoquinoline | |
---|---|---|
Source | PubChem | |
URL | https://pubchem.ncbi.nlm.nih.gov | |
Description | Data deposited in or computed by PubChem | |
InChI |
InChI=1S/C11H11N/c1-8-5-9(2)11-7-12-4-3-10(11)6-8/h3-7H,1-2H3 | |
Source | PubChem | |
URL | https://pubchem.ncbi.nlm.nih.gov | |
Description | Data deposited in or computed by PubChem | |
InChI Key |
KAXQBGPFESZQIL-UHFFFAOYSA-N | |
Source | PubChem | |
URL | https://pubchem.ncbi.nlm.nih.gov | |
Description | Data deposited in or computed by PubChem | |
Canonical SMILES |
CC1=CC(=C2C=NC=CC2=C1)C | |
Source | PubChem | |
URL | https://pubchem.ncbi.nlm.nih.gov | |
Description | Data deposited in or computed by PubChem | |
Molecular Formula |
C11H11N | |
Source | PubChem | |
URL | https://pubchem.ncbi.nlm.nih.gov | |
Description | Data deposited in or computed by PubChem | |
Molecular Weight |
157.21 g/mol | |
Source | PubChem | |
URL | https://pubchem.ncbi.nlm.nih.gov | |
Description | Data deposited in or computed by PubChem | |
Retrosynthesis Analysis
AI-Powered Synthesis Planning: Our tool employs the Template_relevance Pistachio, Template_relevance Bkms_metabolic, Template_relevance Pistachio_ringbreaker, Template_relevance Reaxys, Template_relevance Reaxys_biocatalysis model, leveraging a vast database of chemical reactions to predict feasible synthetic routes.
One-Step Synthesis Focus: Specifically designed for one-step synthesis, it provides concise and direct routes for your target compounds, streamlining the synthesis process.
Accurate Predictions: Utilizing the extensive PISTACHIO, BKMS_METABOLIC, PISTACHIO_RINGBREAKER, REAXYS, REAXYS_BIOCATALYSIS database, our tool offers high-accuracy predictions, reflecting the latest in chemical research and data.
Strategy Settings
Precursor scoring | Relevance Heuristic |
---|---|
Min. plausibility | 0.01 |
Model | Template_relevance |
Template Set | Pistachio/Bkms_metabolic/Pistachio_ringbreaker/Reaxys/Reaxys_biocatalysis |
Top-N result to add to graph | 6 |
Feasible Synthetic Routes
Disclaimer and Information on In-Vitro Research Products
Please be aware that all articles and product information presented on BenchChem are intended solely for informational purposes. The products available for purchase on BenchChem are specifically designed for in-vitro studies, which are conducted outside of living organisms. In-vitro studies, derived from the Latin term "in glass," involve experiments performed in controlled laboratory settings using cells or tissues. It is important to note that these products are not categorized as medicines or drugs, and they have not received approval from the FDA for the prevention, treatment, or cure of any medical condition, ailment, or disease. We must emphasize that any form of bodily introduction of these products into humans or animals is strictly prohibited by law. It is essential to adhere to these guidelines to ensure compliance with legal and ethical standards in research and experimentation.