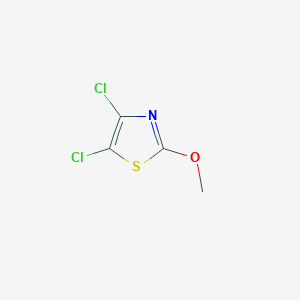
4,5-Dichloro-2-methoxy-1,3-thiazole
- Click on QUICK INQUIRY to receive a quote from our team of experts.
- With the quality product at a COMPETITIVE price, you can focus more on your research.
Overview
Description
4,5-Dichloro-2-methoxy-1,3-thiazole is a heterocyclic organic compound that belongs to the thiazole family. Thiazoles are characterized by a five-membered ring containing three carbon atoms, one sulfur atom, and one nitrogen atom. This particular compound is distinguished by the presence of two chlorine atoms at the 4 and 5 positions and a methoxy group at the 2 position on the thiazole ring. Thiazoles are known for their diverse biological activities and are found in various natural products and synthetic compounds .
Preparation Methods
Synthetic Routes and Reaction Conditions: The synthesis of 4,5-Dichloro-2-methoxy-1,3-thiazole typically involves the reaction of appropriate precursors under controlled conditions. One common method involves the reaction of 2-chloro-1,3-thiazole with methanol in the presence of a base such as sodium methoxide. The reaction is carried out under reflux conditions to facilitate the substitution of the chlorine atom with the methoxy group .
Industrial Production Methods: Industrial production of this compound may involve large-scale synthesis using similar reaction conditions as described above. The process is optimized for yield and purity, often involving purification steps such as recrystallization or chromatography to isolate the desired product .
Chemical Reactions Analysis
Types of Reactions: 4,5-Dichloro-2-methoxy-1,3-thiazole undergoes various chemical reactions, including:
Substitution Reactions: The chlorine atoms at the 4 and 5 positions can be substituted with other nucleophiles such as amines or thiols.
Oxidation and Reduction Reactions: The thiazole ring can undergo oxidation to form sulfoxides or sulfones, and reduction to form dihydrothiazoles.
Cyclization Reactions: The compound can participate in cyclization reactions to form fused ring systems.
Common Reagents and Conditions:
Nucleophilic Substitution: Reagents such as sodium methoxide, amines, or thiols in polar solvents.
Oxidation: Reagents such as hydrogen peroxide or m-chloroperbenzoic acid.
Reduction: Reagents such as lithium aluminum hydride or sodium borohydride.
Major Products:
Substituted Thiazoles: Products with various substituents replacing the chlorine atoms.
Sulfoxides and Sulfones: Products of oxidation reactions.
Dihydrothiazoles: Products of reduction reactions.
Scientific Research Applications
4,5-Dichloro-2-methoxy-1,3-thiazole has a wide range of applications in scientific research:
Chemistry: Used as a building block for the synthesis of more complex thiazole derivatives.
Biology: Investigated for its potential biological activities, including antimicrobial, antifungal, and anticancer properties.
Medicine: Explored as a potential lead compound for drug development due to its diverse biological activities.
Industry: Utilized in the production of agrochemicals, dyes, and other industrial chemicals
Mechanism of Action
The mechanism of action of 4,5-Dichloro-2-methoxy-1,3-thiazole involves its interaction with various molecular targets and pathways. The compound can act as an inhibitor of specific enzymes or receptors, leading to its biological effects. For example, it may inhibit microbial enzymes, leading to antimicrobial activity, or interact with cellular pathways involved in cancer cell proliferation .
Comparison with Similar Compounds
4,5-Dichloro-1,3-thiazole: Lacks the methoxy group, which may affect its reactivity and biological activity.
2-Methoxy-1,3-thiazole: Lacks the chlorine atoms, which may influence its chemical properties and applications.
4,5-Dichloro-2-methyl-1,3-thiazole: Contains a methyl group instead of a methoxy group, leading to differences in solubility and reactivity
Uniqueness: 4,5-Dichloro-2-methoxy-1,3-thiazole is unique due to the presence of both chlorine atoms and a methoxy group, which confer specific chemical properties and biological activities. This combination of substituents makes it a valuable compound for various applications in research and industry .
Properties
CAS No. |
62019-52-7 |
---|---|
Molecular Formula |
C4H3Cl2NOS |
Molecular Weight |
184.04 g/mol |
IUPAC Name |
4,5-dichloro-2-methoxy-1,3-thiazole |
InChI |
InChI=1S/C4H3Cl2NOS/c1-8-4-7-2(5)3(6)9-4/h1H3 |
InChI Key |
IRBCEGBDYMWTTP-UHFFFAOYSA-N |
Canonical SMILES |
COC1=NC(=C(S1)Cl)Cl |
Origin of Product |
United States |
Disclaimer and Information on In-Vitro Research Products
Please be aware that all articles and product information presented on BenchChem are intended solely for informational purposes. The products available for purchase on BenchChem are specifically designed for in-vitro studies, which are conducted outside of living organisms. In-vitro studies, derived from the Latin term "in glass," involve experiments performed in controlled laboratory settings using cells or tissues. It is important to note that these products are not categorized as medicines or drugs, and they have not received approval from the FDA for the prevention, treatment, or cure of any medical condition, ailment, or disease. We must emphasize that any form of bodily introduction of these products into humans or animals is strictly prohibited by law. It is essential to adhere to these guidelines to ensure compliance with legal and ethical standards in research and experimentation.