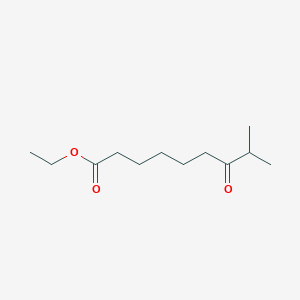
Ethyl-8-methyl-7-oxononanoate
Overview
Description
Ethyl-8-methyl-7-oxononanoate is a chemical compound with the molecular formula C12H22O3 . It is known for its unique structure, which consists of 12 carbon atoms, 22 hydrogen atoms, and 3 oxygen atoms. This compound is used in various scientific research applications due to its distinctive properties.
Preparation Methods
Synthetic Routes and Reaction Conditions: Ethyl-8-methyl-7-oxononanoate can be synthesized through several routes. One common method involves the esterification of 8-methyl-7-oxononanoic acid with ethanol under acidic conditions . The reaction typically requires a catalyst such as sulfuric acid and is carried out at elevated temperatures to drive the reaction to completion.
Industrial Production Methods: In industrial settings, the production of this compound often involves continuous flow processes to ensure high yield and purity. The use of advanced catalysts and optimized reaction conditions helps in achieving efficient production .
Chemical Reactions Analysis
Types of Reactions: Ethyl-8-methyl-7-oxononanoate undergoes various chemical reactions, including:
Oxidation: This compound can be oxidized to form corresponding carboxylic acids.
Reduction: Reduction reactions can convert the ketone group to an alcohol.
Substitution: The ester group can undergo nucleophilic substitution reactions.
Common Reagents and Conditions:
Oxidation: Common oxidizing agents include potassium permanganate and chromium trioxide.
Reduction: Reducing agents such as lithium aluminum hydride or sodium borohydride are typically used.
Substitution: Nucleophiles like amines or alcohols can be used under basic or acidic conditions.
Major Products:
Oxidation: 8-methyl-7-oxononanoic acid.
Reduction: Ethyl-8-methyl-7-hydroxynonanoate.
Substitution: Various substituted esters depending on the nucleophile used.
Scientific Research Applications
Ethyl-8-methyl-7-oxononanoate has a wide range of applications in scientific research:
Chemistry: Used as an intermediate in organic synthesis and as a reagent in various chemical reactions.
Biology: Studied for its potential biological activities and interactions with biomolecules.
Medicine: Investigated for its potential therapeutic properties and as a precursor in drug synthesis.
Industry: Utilized in the production of specialty chemicals and materials.
Mechanism of Action
The mechanism of action of ethyl-8-methyl-7-oxononanoate involves its interaction with specific molecular targets and pathways. It is known to participate in enzymatic reactions where it acts as a substrate or inhibitor . The compound’s effects are mediated through its ability to form covalent bonds with target molecules, leading to changes in their activity and function .
Comparison with Similar Compounds
- 8-oxooctanoate: A similar compound with one less carbon atom.
- 9-oxononanoate: A similar compound with a different substitution pattern .
Ethyl-8-methyl-7-oxononanoate stands out due to its unique combination of functional groups, making it a valuable compound in various scientific and industrial applications.
Properties
IUPAC Name |
ethyl 8-methyl-7-oxononanoate | |
---|---|---|
Source | PubChem | |
URL | https://pubchem.ncbi.nlm.nih.gov | |
Description | Data deposited in or computed by PubChem | |
InChI |
InChI=1S/C12H22O3/c1-4-15-12(14)9-7-5-6-8-11(13)10(2)3/h10H,4-9H2,1-3H3 | |
Source | PubChem | |
URL | https://pubchem.ncbi.nlm.nih.gov | |
Description | Data deposited in or computed by PubChem | |
InChI Key |
MDUYTVHVDUOISU-UHFFFAOYSA-N | |
Source | PubChem | |
URL | https://pubchem.ncbi.nlm.nih.gov | |
Description | Data deposited in or computed by PubChem | |
Canonical SMILES |
CCOC(=O)CCCCCC(=O)C(C)C | |
Source | PubChem | |
URL | https://pubchem.ncbi.nlm.nih.gov | |
Description | Data deposited in or computed by PubChem | |
Molecular Formula |
C12H22O3 | |
Source | PubChem | |
URL | https://pubchem.ncbi.nlm.nih.gov | |
Description | Data deposited in or computed by PubChem | |
DSSTOX Substance ID |
DTXSID90560027 | |
Record name | Ethyl 8-methyl-7-oxononanoate | |
Source | EPA DSSTox | |
URL | https://comptox.epa.gov/dashboard/DTXSID90560027 | |
Description | DSSTox provides a high quality public chemistry resource for supporting improved predictive toxicology. | |
Molecular Weight |
214.30 g/mol | |
Source | PubChem | |
URL | https://pubchem.ncbi.nlm.nih.gov | |
Description | Data deposited in or computed by PubChem | |
CAS No. |
126245-80-5 | |
Record name | Ethyl 8-methyl-7-oxononanoate | |
Source | EPA DSSTox | |
URL | https://comptox.epa.gov/dashboard/DTXSID90560027 | |
Description | DSSTox provides a high quality public chemistry resource for supporting improved predictive toxicology. | |
Retrosynthesis Analysis
AI-Powered Synthesis Planning: Our tool employs the Template_relevance Pistachio, Template_relevance Bkms_metabolic, Template_relevance Pistachio_ringbreaker, Template_relevance Reaxys, Template_relevance Reaxys_biocatalysis model, leveraging a vast database of chemical reactions to predict feasible synthetic routes.
One-Step Synthesis Focus: Specifically designed for one-step synthesis, it provides concise and direct routes for your target compounds, streamlining the synthesis process.
Accurate Predictions: Utilizing the extensive PISTACHIO, BKMS_METABOLIC, PISTACHIO_RINGBREAKER, REAXYS, REAXYS_BIOCATALYSIS database, our tool offers high-accuracy predictions, reflecting the latest in chemical research and data.
Strategy Settings
Precursor scoring | Relevance Heuristic |
---|---|
Min. plausibility | 0.01 |
Model | Template_relevance |
Template Set | Pistachio/Bkms_metabolic/Pistachio_ringbreaker/Reaxys/Reaxys_biocatalysis |
Top-N result to add to graph | 6 |
Feasible Synthetic Routes
Disclaimer and Information on In-Vitro Research Products
Please be aware that all articles and product information presented on BenchChem are intended solely for informational purposes. The products available for purchase on BenchChem are specifically designed for in-vitro studies, which are conducted outside of living organisms. In-vitro studies, derived from the Latin term "in glass," involve experiments performed in controlled laboratory settings using cells or tissues. It is important to note that these products are not categorized as medicines or drugs, and they have not received approval from the FDA for the prevention, treatment, or cure of any medical condition, ailment, or disease. We must emphasize that any form of bodily introduction of these products into humans or animals is strictly prohibited by law. It is essential to adhere to these guidelines to ensure compliance with legal and ethical standards in research and experimentation.