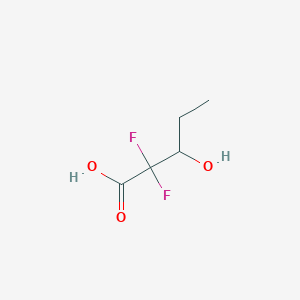
2,2-Difluoro-3-hydroxypentanoic acid
Overview
Description
2,2-Difluoro-3-hydroxypentanoic acid is an organic compound with the molecular formula C5H8F2O3 and a molecular weight of 154.11 g/mol It is characterized by the presence of two fluorine atoms and a hydroxyl group attached to a pentanoic acid backbone
Biochemical Analysis
Biochemical Properties
2,2-Difluoro-3-hydroxypentanoic acid plays a significant role in biochemical reactions, particularly in the context of enzyme interactions. It is known to interact with enzymes involved in metabolic pathways, potentially acting as an inhibitor or substrate. The hydroxyl group in this compound allows it to form hydrogen bonds with active sites of enzymes, influencing their activity. Additionally, the presence of fluorine atoms can affect the compound’s reactivity and interaction with biomolecules .
Cellular Effects
This compound has been observed to influence various cellular processes. It can affect cell signaling pathways, gene expression, and cellular metabolism. The compound’s interaction with enzymes and proteins within the cell can lead to alterations in metabolic flux and energy production. Furthermore, this compound may impact cell proliferation and apoptosis, depending on the concentration and exposure duration .
Molecular Mechanism
The molecular mechanism of this compound involves its interaction with specific enzymes and proteins. The compound can bind to enzyme active sites, either inhibiting or activating their function. This binding can lead to changes in enzyme conformation and activity, ultimately affecting metabolic pathways. Additionally, this compound may influence gene expression by interacting with transcription factors or other regulatory proteins .
Temporal Effects in Laboratory Settings
In laboratory settings, the effects of this compound can vary over time. The compound’s stability and degradation are important factors to consider. Studies have shown that this compound is relatively stable under standard laboratory conditions, but its effects on cellular function can change with prolonged exposure. Long-term studies have indicated potential cumulative effects on cellular metabolism and gene expression .
Dosage Effects in Animal Models
The effects of this compound in animal models are dose-dependent. At lower doses, the compound may exhibit minimal toxicity and can be used to study its biochemical properties. At higher doses, this compound may cause adverse effects, including toxicity and disruption of normal cellular functions. It is crucial to determine the appropriate dosage to avoid potential harmful effects in animal studies .
Metabolic Pathways
This compound is involved in various metabolic pathways. It can be metabolized by enzymes such as dehydrogenases and oxidases, leading to the formation of intermediate metabolites. These metabolites can further participate in biochemical reactions, influencing metabolic flux and the levels of other metabolites. The presence of fluorine atoms in the compound can also affect its metabolic stability and reactivity .
Transport and Distribution
The transport and distribution of this compound within cells and tissues are mediated by specific transporters and binding proteins. The compound can be taken up by cells through active transport mechanisms and distributed to various cellular compartments. Its localization and accumulation within cells can influence its biochemical activity and interactions with other biomolecules .
Subcellular Localization
This compound exhibits specific subcellular localization patterns. It can be targeted to specific compartments or organelles within the cell, depending on its chemical properties and interactions with targeting signals or post-translational modifications. The subcellular localization of this compound can affect its activity and function, influencing cellular processes and metabolic pathways .
Preparation Methods
Synthetic Routes and Reaction Conditions
One common method is the fluorination of 3-hydroxypentanoic acid using a fluorinating agent such as diethylaminosulfur trifluoride (DAST) under controlled conditions . The reaction is usually carried out in an inert atmosphere to prevent unwanted side reactions.
Industrial Production Methods
Industrial production of 2,2-Difluoro-3-hydroxypentanoic acid may involve large-scale fluorination processes using specialized equipment to handle the reactive fluorinating agents safely. The process parameters, such as temperature, pressure, and reaction time, are optimized to maximize yield and purity while minimizing by-products.
Chemical Reactions Analysis
Types of Reactions
2,2-Difluoro-3-hydroxypentanoic acid can undergo various chemical reactions, including:
Oxidation: The hydroxyl group can be oxidized to form a ketone or carboxylic acid derivative.
Reduction: The compound can be reduced to form a difluorinated alcohol.
Substitution: The fluorine atoms can be substituted with other functional groups under appropriate conditions.
Common Reagents and Conditions
Oxidation: Common oxidizing agents include potassium permanganate (KMnO4) and chromium trioxide (CrO3).
Reduction: Reducing agents such as lithium aluminum hydride (LiAlH4) or sodium borohydride (NaBH4) are used.
Substitution: Nucleophilic substitution reactions can be carried out using reagents like sodium methoxide (NaOCH3) or potassium tert-butoxide (KOtBu).
Major Products Formed
Oxidation: Formation of 2,2-difluoro-3-oxopentanoic acid or 2,2-difluoropentanoic acid.
Reduction: Formation of 2,2-difluoro-3-hydroxypentanol.
Substitution: Formation of various substituted pentanoic acid derivatives depending on the nucleophile used.
Scientific Research Applications
2,2-Difluoro-3-hydroxypentanoic acid has several applications in scientific research:
Chemistry: Used as a building block for the synthesis of more complex fluorinated compounds.
Biology: Studied for its potential effects on biological systems, including enzyme inhibition and metabolic pathways.
Medicine: Investigated for its potential use in drug development, particularly in designing fluorinated pharmaceuticals with improved bioavailability and metabolic stability.
Mechanism of Action
The mechanism of action of 2,2-Difluoro-3-hydroxypentanoic acid involves its interaction with molecular targets such as enzymes and receptors. The presence of fluorine atoms can enhance the compound’s binding affinity and selectivity for specific targets, leading to altered biological activity. The hydroxyl group can participate in hydrogen bonding, further influencing the compound’s interactions with biological molecules .
Comparison with Similar Compounds
Similar Compounds
2,2-Difluoro-3-hydroxybutanoic acid: Similar structure but with a shorter carbon chain.
2,2-Difluoro-3-hydroxyhexanoic acid: Similar structure but with a longer carbon chain.
2,2-Difluoro-3-hydroxypropanoic acid: Similar structure but with an even shorter carbon chain.
Uniqueness
2,2-Difluoro-3-hydroxypentanoic acid is unique due to its specific carbon chain length and the positioning of the fluorine atoms and hydroxyl group. These structural features confer distinct chemical and biological properties, making it a valuable compound for various applications .
Biological Activity
2,2-Difluoro-3-hydroxypentanoic acid (DFHPA) is a fluorinated compound that has garnered attention in biochemical research due to its unique structural properties and potential biological activities. This article delves into the compound's biochemical interactions, cellular effects, mechanisms of action, and its implications in scientific research.
DFHPA is characterized by its ability to interact with various enzymes involved in metabolic pathways. The presence of the hydroxyl group allows for hydrogen bonding with enzyme active sites, which can modulate enzyme activity either as an inhibitor or substrate. The fluorine atoms contribute to the compound's reactivity, influencing its interactions with biomolecules.
Property | Description |
---|---|
Molecular Formula | CHFO |
Role in Metabolism | Acts as an enzyme inhibitor or substrate |
Interaction Mechanism | Forms hydrogen bonds with active sites of enzymes |
Stability | Relatively stable under standard laboratory conditions |
Cellular Effects
DFHPA significantly influences cellular processes, including cell signaling pathways and gene expression. Its interactions with enzymes can alter metabolic flux and energy production. Notably, DFHPA has been shown to impact cell proliferation and apoptosis, depending on concentration and exposure duration.
Case Study: Dose-Dependent Effects in Animal Models
In research involving animal models, DFHPA exhibited dose-dependent effects. At lower doses, it demonstrated minimal toxicity and facilitated the exploration of its biochemical properties. Conversely, higher doses led to adverse effects, indicating the importance of dosage in determining the compound's safety profile.
Molecular Mechanism
The mechanism of action for DFHPA involves binding to specific enzymes and proteins. This binding can lead to conformational changes in enzyme activity, thereby affecting metabolic pathways. Additionally, DFHPA may influence gene expression by interacting with transcription factors.
Table 2: Summary of Molecular Mechanisms
Mechanism | Description |
---|---|
Enzyme Interaction | Binds to active sites; alters conformation and activity |
Gene Expression Modulation | Interacts with transcription factors; influences gene regulation |
Metabolic Pathways
DFHPA participates in various metabolic pathways through its metabolism by enzymes such as dehydrogenases and oxidases. The fluorine atoms enhance the compound's metabolic stability, leading to the formation of intermediate metabolites that further engage in biochemical reactions.
Transport and Distribution
The transport of DFHPA within biological systems is mediated by specific transporters. It can be taken up by cells through active transport mechanisms and distributed across cellular compartments, influencing its biological activity.
Scientific Research Applications
DFHPA has multiple applications in scientific research:
- Chemistry : Serves as a building block for synthesizing complex fluorinated compounds.
- Biology : Investigated for its effects on enzyme inhibition and metabolic pathways.
- Medicine : Explored for potential use in drug development, particularly for designing fluorinated pharmaceuticals with enhanced bioavailability.
- Industry : Utilized in producing specialty chemicals with unique properties due to fluorination .
Properties
IUPAC Name |
2,2-difluoro-3-hydroxypentanoic acid | |
---|---|---|
Source | PubChem | |
URL | https://pubchem.ncbi.nlm.nih.gov | |
Description | Data deposited in or computed by PubChem | |
InChI |
InChI=1S/C5H8F2O3/c1-2-3(8)5(6,7)4(9)10/h3,8H,2H2,1H3,(H,9,10) | |
Source | PubChem | |
URL | https://pubchem.ncbi.nlm.nih.gov | |
Description | Data deposited in or computed by PubChem | |
InChI Key |
RLFXUHFTSMNNLS-UHFFFAOYSA-N | |
Source | PubChem | |
URL | https://pubchem.ncbi.nlm.nih.gov | |
Description | Data deposited in or computed by PubChem | |
Canonical SMILES |
CCC(C(C(=O)O)(F)F)O | |
Source | PubChem | |
URL | https://pubchem.ncbi.nlm.nih.gov | |
Description | Data deposited in or computed by PubChem | |
Molecular Formula |
C5H8F2O3 | |
Source | PubChem | |
URL | https://pubchem.ncbi.nlm.nih.gov | |
Description | Data deposited in or computed by PubChem | |
DSSTOX Substance ID |
DTXSID40710217 | |
Record name | 2,2-Difluoro-3-hydroxypentanoic acid | |
Source | EPA DSSTox | |
URL | https://comptox.epa.gov/dashboard/DTXSID40710217 | |
Description | DSSTox provides a high quality public chemistry resource for supporting improved predictive toxicology. | |
Molecular Weight |
154.11 g/mol | |
Source | PubChem | |
URL | https://pubchem.ncbi.nlm.nih.gov | |
Description | Data deposited in or computed by PubChem | |
CAS No. |
1233233-76-5 | |
Record name | 2,2-Difluoro-3-hydroxypentanoic acid | |
Source | EPA DSSTox | |
URL | https://comptox.epa.gov/dashboard/DTXSID40710217 | |
Description | DSSTox provides a high quality public chemistry resource for supporting improved predictive toxicology. | |
Record name | 2,2-difluoro-3-hydroxypentanoic acid | |
Source | European Chemicals Agency (ECHA) | |
URL | https://echa.europa.eu/information-on-chemicals | |
Description | The European Chemicals Agency (ECHA) is an agency of the European Union which is the driving force among regulatory authorities in implementing the EU's groundbreaking chemicals legislation for the benefit of human health and the environment as well as for innovation and competitiveness. | |
Explanation | Use of the information, documents and data from the ECHA website is subject to the terms and conditions of this Legal Notice, and subject to other binding limitations provided for under applicable law, the information, documents and data made available on the ECHA website may be reproduced, distributed and/or used, totally or in part, for non-commercial purposes provided that ECHA is acknowledged as the source: "Source: European Chemicals Agency, http://echa.europa.eu/". Such acknowledgement must be included in each copy of the material. ECHA permits and encourages organisations and individuals to create links to the ECHA website under the following cumulative conditions: Links can only be made to webpages that provide a link to the Legal Notice page. | |
Synthesis routes and methods
Procedure details
Retrosynthesis Analysis
AI-Powered Synthesis Planning: Our tool employs the Template_relevance Pistachio, Template_relevance Bkms_metabolic, Template_relevance Pistachio_ringbreaker, Template_relevance Reaxys, Template_relevance Reaxys_biocatalysis model, leveraging a vast database of chemical reactions to predict feasible synthetic routes.
One-Step Synthesis Focus: Specifically designed for one-step synthesis, it provides concise and direct routes for your target compounds, streamlining the synthesis process.
Accurate Predictions: Utilizing the extensive PISTACHIO, BKMS_METABOLIC, PISTACHIO_RINGBREAKER, REAXYS, REAXYS_BIOCATALYSIS database, our tool offers high-accuracy predictions, reflecting the latest in chemical research and data.
Strategy Settings
Precursor scoring | Relevance Heuristic |
---|---|
Min. plausibility | 0.01 |
Model | Template_relevance |
Template Set | Pistachio/Bkms_metabolic/Pistachio_ringbreaker/Reaxys/Reaxys_biocatalysis |
Top-N result to add to graph | 6 |
Feasible Synthetic Routes
Disclaimer and Information on In-Vitro Research Products
Please be aware that all articles and product information presented on BenchChem are intended solely for informational purposes. The products available for purchase on BenchChem are specifically designed for in-vitro studies, which are conducted outside of living organisms. In-vitro studies, derived from the Latin term "in glass," involve experiments performed in controlled laboratory settings using cells or tissues. It is important to note that these products are not categorized as medicines or drugs, and they have not received approval from the FDA for the prevention, treatment, or cure of any medical condition, ailment, or disease. We must emphasize that any form of bodily introduction of these products into humans or animals is strictly prohibited by law. It is essential to adhere to these guidelines to ensure compliance with legal and ethical standards in research and experimentation.