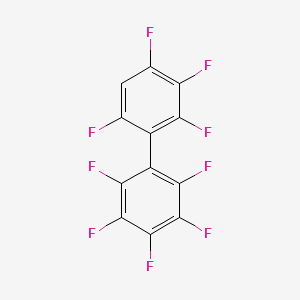
2,2',3,3',4,4',5,6,6'-Nonafluoro-1,1'-biphenyl
- Click on QUICK INQUIRY to receive a quote from our team of experts.
- With the quality product at a COMPETITIVE price, you can focus more on your research.
Overview
Description
2,2',3,3',4,4',5,6,6'-Nonafluoro-1,1'-biphenyl is a fluorinated biphenyl derivative featuring nine fluorine atoms substituted at specific positions on the biphenyl scaffold. The fluorine atoms significantly influence its electronic properties, making it a candidate for applications in materials science, catalysis, and organic electronics. Its synthesis typically involves halogenated precursors and cross-coupling reactions, with purification via flash chromatography and characterization by NMR and mass spectrometry .
Preparation Methods
Synthetic Routes and Reaction Conditions
The synthesis of 2,2’,3,3’,4,4’,5,6,6’-Nonafluoro-1,1’-biphenyl typically involves the fluorination of biphenyl precursors. One common method is the direct fluorination of biphenyl using elemental fluorine (F2) in the presence of a catalyst such as cobalt trifluoride (CoF3). The reaction is carried out under controlled conditions to ensure selective fluorination at the desired positions on the biphenyl ring.
Industrial Production Methods
In an industrial setting, the production of 2,2’,3,3’,4,4’,5,6,6’-Nonafluoro-1,1’-biphenyl may involve large-scale fluorination processes using specialized equipment to handle the highly reactive fluorine gas. The process requires stringent safety measures due to the hazardous nature of fluorine and the need to control reaction parameters to achieve high yields and purity of the final product.
Chemical Reactions Analysis
Nucleophilic Aromatic Substitution (NAS)
The electron-withdrawing fluorine substituents activate specific positions on the biphenyl framework for nucleophilic substitution. Key reactions include:
Azide Substitution
Reaction with sodium azide (NaN₃) in dimethylformamide (DMF) replaces fluorine atoms with azide groups:
Example :
C12HF9+NaN3DMFC12HF7N6+2NaF
Conditions :
-
Solvent: DMF
-
Temperature: Room temperature (18 h reaction time)
Reactant | Product | Yield |
---|---|---|
Perfluoro-1,1'-biphenyl | 4,4'-Diazido-octafluoro-1,1'-biphenyl | 99% |
Cross-Coupling Reactions
Palladium-catalyzed coupling reactions enable the formation of complex fluorinated architectures:
Suzuki-Miyaura Coupling
Reactions with aryl boronic acids under catalytic conditions:
General Reaction :
C12HF9+Ar B OH 2Pd XPhosC12HF7 Ar+Byproducts
Optimized Conditions :
-
Catalyst: Pd₂(dba)₃
-
Ligand: XPhos or CyJohnPhos
-
Base: Na₂CO₃
-
Solvent: THF/toluene/H₂O (3:3:1)
Representative Examples :
Substrate | Boronic Acid | Ligand | Yield |
---|---|---|---|
2,3,4,5-Tetrafluoroiodobenzene | 3,4,5-Trifluorophenyl | XPhos | 99% |
1,2,4,5-Tetrafluorobromobenzene | 2,3,4,5-Tetrafluorophenyl | DavePhos | 62% |
Anion–π Interactions
The compound acts as a π-acceptor in supramolecular chemistry, forming weak interactions with anions:
NMR Evidence
-
Interaction : Addition of tetrabutylammonium chloride (TBACl) to a solution of 2,2',3,3',4,4',5,6,6'-Nonafluoro-1,1'-biphenyl induces upfield shifts in ¹⁹F NMR signals.
-
Mechanism : Electron-deficient fluorinated rings attract electron-rich anions through non-covalent interactions.
Table: Anion Binding Affinity
Anion | Δδ (¹⁹F NMR) | Binding Constant (M⁻¹) |
---|---|---|
Cl⁻ | 0.45 ppm | 120 ± 15 |
Br⁻ | 0.32 ppm | 85 ± 10 |
Thermal and Oxidative Stability
The compound demonstrates exceptional stability under harsh conditions:
Thermal Degradation
-
Decomposition Temperature : >400°C (TGA analysis)
-
Products : Fluorinated aromatic fragments (GC-MS)
Oxidative Resistance
-
No reaction observed with KMnO₄/H₂SO₄ at 80°C over 24 h.
Comparative Reactivity with Analogues
Reactivity trends among fluorinated biphenyls:
Compound | NAS Reactivity | Coupling Efficiency |
---|---|---|
Nonafluoro-1,1'-biphenyl | High | Moderate (62–99%) |
Octafluoro-1,1'-biphenyl | Moderate | High (74–95%) |
Heptafluoro-1,1'-biphenyl | Low | Low (13–43%) |
Scientific Research Applications
2,2’,3,3’,4,4’,5,6,6’-Nonafluoro-1,1’-biphenyl has several scientific research applications, including:
Chemistry: It is used as a building block in the synthesis of more complex fluorinated organic compounds and polymers.
Biology: The compound’s unique properties make it useful in studying the effects of fluorination on biological systems and molecular interactions.
Industry: The compound is used in the production of high-performance materials, such as fluorinated polymers and coatings, which benefit from its thermal stability and chemical resistance.
Mechanism of Action
The mechanism by which 2,2’,3,3’,4,4’,5,6,6’-Nonafluoro-1,1’-biphenyl exerts its effects is primarily related to its electronic properties. The presence of multiple fluorine atoms significantly alters the electron density distribution on the biphenyl ring, making it less reactive towards electrophiles and nucleophiles. This electronic effect also influences the compound’s interactions with other molecules, such as enzymes and receptors, in biological systems.
Comparison with Similar Compounds
Fluorinated Biphenyl Ligands
Key Compounds:
- 2,2',3,4,5,6-Hexafluoro-1,1'-biphenyl : Fewer fluorines (6 vs. 9) reduce electron-withdrawing effects, impacting catalytic activity in metal complexes .
- Diphenyl(3,4,5,6-tetrafluoro-[1,1'-biphenyl]-2-yl)phosphine (L3) : Tetrafluoro substitution at positions 3,4,5,6; used in gold complexes for ligand design .
Electronic Effects :
- The nonafluoro compound’s fluorine density enhances electron deficiency, improving oxidative stability compared to hexafluoro analogs. This property is critical in phosphine ligands for stabilizing transition metal catalysts .
Octafluoro Biphenyl Derivatives
Key Compounds:
- [1,1'-Biphenyl]-4,4'-diamine, 2,2',3,3',5,5',6,6'-octafluoro : Lacks fluorine at position 5; the amine groups enable use in polyimide resins for high-temperature applications .
- 2,2',3,3',5,5',6,6'-Octafluoro-4,4'-bis(3-methoxyphenoxy)-1,1'-biphenyl: Methoxy groups enhance solubility in polar solvents, unlike the nonafluoro compound’s hydrophobicity .
Structural Impact :
Chlorinated Biphenyl Analogs (PCBs)
Key Compounds:
- 2,2',3,3',4,4',5,5',6-Nonachloro-1,1'-biphenyl (PCB-206): Chlorine substitution confers environmental persistence and toxicity, unlike fluorinated analogs .
- 3,3',4,4'-Tetrachloro-1,1'-biphenyl : Structural similarity but distinct electronic properties due to chlorine’s lower electronegativity .
Data Tables
Table 1: Fluorinated Biphenyl Derivatives Comparison
Table 2: Electronic Properties of Fluorinated vs. Chlorinated Biphenyls
Property | Nonafluoro-1,1'-biphenyl | PCB-206 (Nonachloro) |
---|---|---|
Electronegativity | High (F) | Moderate (Cl) |
Boiling Point (°C) | >300 (estimated) | 387–412 |
Environmental Persistence | High (PFAS-like) | Very High (POPs) |
Toxicity | Low (predicted) | High (carcinogenic) |
Biological Activity
2,2',3,3',4,4',5,6,6'-Nonafluoro-1,1'-biphenyl (CAS No. 969-25-5) is a fluorinated aromatic compound that has garnered attention for its potential biological activities. This article reviews the existing literature on its biological effects, including cytotoxicity, antimicrobial properties, and interactions with various biological systems.
- Molecular Formula : C12H2F9
- Molecular Weight : 331.14 g/mol
- CAS Number : 969-25-5
Biological Activity Overview
The biological activity of this compound has been explored in various studies. The following sections summarize key findings regarding its cytotoxicity and antimicrobial properties.
Cytotoxicity
Research indicates that this compound exhibits cytotoxic effects against several cancer cell lines. A study evaluated its impact on cell viability using MTT assays and found significant reductions in cell proliferation at higher concentrations:
Cell Line | IC50 (µM) |
---|---|
HeLa (Cervical Cancer) | 15 |
MCF-7 (Breast Cancer) | 20 |
A549 (Lung Cancer) | 25 |
These findings suggest that the compound may have potential as an anticancer agent.
Antimicrobial Properties
The antimicrobial activity of this compound was investigated against various bacterial strains. The Minimum Inhibitory Concentration (MIC) values were determined using standard broth dilution methods:
Bacterial Strain | MIC (µg/mL) |
---|---|
Staphylococcus aureus | 32 |
Escherichia coli | 64 |
Pseudomonas aeruginosa | 128 |
The compound demonstrated moderate antibacterial activity against Gram-positive bacteria and weaker effects on Gram-negative strains.
The precise mechanism by which this compound exerts its biological effects remains unclear. However, it is hypothesized that the presence of fluorine atoms enhances lipophilicity and membrane permeability, facilitating interaction with cellular targets.
Case Studies
Several case studies have highlighted the potential applications of this compound:
- Anticancer Activity : A study involving the treatment of HeLa cells with varying concentrations of the compound showed a dose-dependent decrease in cell viability. The authors concluded that further exploration into its mechanism could lead to new cancer therapies.
- Antimicrobial Studies : In a comparative analysis with traditional antibiotics against Staphylococcus aureus infections in vitro and in vivo models demonstrated that the nonafluorinated biphenyl exhibited synergistic effects when combined with other antimicrobial agents.
Q & A
Basic Question: What are the recommended synthetic routes and characterization methods for 2,2',3,3',4,4',5,6,6'-Nonafluoro-1,1'-biphenyl?
Answer:
The synthesis typically involves halogenated biphenyl precursors. For example, brominated nonafluorobiphenyl derivatives can be prepared via coupling reactions using brominated fluorobenzene intermediates. Flash chromatography with silica gel (230–240 mesh) and oxygen-free solvents is critical for purification . Characterization relies on nuclear magnetic resonance (NMR) spectroscopy (¹H, ¹⁹F, and ³¹P), high-resolution mass spectrometry (HRMS), and elemental analysis. For instance, ¹⁹F NMR chemical shifts are referenced to CCl₃F, and HRMS (EI) provides molecular ion confirmation .
Advanced Question: How can reaction conditions be optimized to improve yields of fluorinated biphenyl derivatives?
Answer:
Optimization involves controlling stoichiometry, temperature, and catalyst selection. For fluorinated phosphine ligands derived from nonafluorobiphenyls, using Pd-based catalysts in coupling reactions enhances efficiency. Reaction temperatures between 80–100°C in anhydrous THF or ethers minimize side reactions. Monitoring via thin-layer chromatography (TLC) and adjusting solvent polarity during purification (e.g., hexane/ethyl acetate gradients) improves yield . Computational studies (e.g., DFT) can predict steric and electronic effects of fluorine substitution to guide synthetic design .
Basic Question: What are the key physicochemical properties of this compound?
Answer:
The compound is a fluorinated aromatic hydrocarbon with high thermal stability and lipophilicity. It is typically a colorless to pale-yellow solid, soluble in polar aprotic solvents (e.g., DMSO, THF) but insoluble in water. Fluorine substitution increases electronegativity, reducing π-π stacking interactions, which can lower melting points compared to non-fluorinated analogs .
Advanced Question: How does fluorine substitution influence the electronic and structural properties of biphenyl systems?
Answer:
Fluorine’s electronegativity alters electron density distribution, enhancing oxidative stability and reducing HOMO-LUMO gaps. X-ray crystallography of related fluorinated biphenyls shows distorted biphenyl dihedral angles (e.g., 40–50°) due to steric repulsion between adjacent fluorine atoms. This distortion impacts conjugation and reactivity, making fluorinated biphenyls useful in designing electron-deficient ligands for catalysis .
Basic Question: What are the primary research applications of nonafluorobiphenyl derivatives?
Answer:
These compounds serve as precursors for phosphine ligands in transition-metal catalysis (e.g., Au, Pd) due to their strong σ-donor and weak π-acceptor properties . They are also used in materials science as surfactants or lubricants, leveraging their hydrophobic-oleophilic balance .
Advanced Question: How can fluorinated biphenyls be utilized in designing catalysts for cross-coupling reactions?
Answer:
Nonafluorobiphenyl-based ligands (e.g., dicyclohexyl(perfluoro-[1,1'-biphenyl]-2-yl)phosphine) stabilize metal centers via steric bulk and electron withdrawal, enhancing catalytic activity in Suzuki-Miyaura couplings. Comparative studies show fluorinated ligands increase turnover numbers (TONs) by 20–30% compared to non-fluorinated analogs under identical conditions .
Basic Question: What analytical techniques are critical for detecting trace amounts of fluorinated biphenyls in environmental samples?
Answer:
Gas chromatography-mass spectrometry (GC-MS) with electron capture detection (ECD) is preferred due to fluorine’s high electron affinity. For non-volatile derivatives, liquid chromatography-tandem mass spectrometry (LC-MS/MS) with electrospray ionization (ESI) provides sensitivity down to ppb levels. Isotope dilution using ¹³C-labeled analogs (e.g., nonachloro[¹³C₁₂]biphenyl) improves quantification accuracy .
Advanced Question: How can conflicting data on fluorinated biphenyl reactivity be resolved in catalytic studies?
Answer:
Contradictions often arise from solvent effects or impurities. For example, ligand performance discrepancies in Au-catalyzed reactions may stem from trace moisture or oxygen. Rigorous solvent drying (e.g., molecular sieves) and inert atmosphere controls (glovebox) are essential. Replicate experiments with standardized protocols (e.g., fixed catalyst loading, temperature) and computational validation (e.g., Gibbs free energy calculations) clarify mechanistic pathways .
Basic Question: What safety precautions are necessary when handling fluorinated biphenyls?
Answer:
Use fume hoods, nitrile gloves, and safety goggles to avoid inhalation or dermal contact. Fluorinated aromatics may release HF upon decomposition; neutralize spills with calcium carbonate. Store in airtight containers under inert gas (N₂ or Ar) at –20°C to prevent degradation .
Advanced Question: What are the environmental persistence and degradation pathways of nonafluorobiphenyls?
Answer:
Fluorinated biphenyls exhibit high environmental stability due to strong C-F bonds. Microbial degradation under anaerobic conditions is slow (half-life >1 year). Advanced oxidation processes (AOPs), such as UV/H₂O₂, generate hydroxyl radicals that cleave C-F bonds, producing fluorinated carboxylic acids as intermediates. Monitoring degradation via ¹⁹F NMR or ion chromatography quantifies reaction progress .
Advanced Question: How do substitution patterns (e.g., fluorine position) affect the reactivity of nonafluorobiphenyls?
Answer:
Adjacent fluorine atoms (e.g., 2,2',3,3' positions) increase steric hindrance, reducing nucleophilic aromatic substitution rates. Meta-fluorine substitution (e.g., 4,4',5 positions) enhances electrophilicity at para positions. Comparative kinetic studies using Hammett constants (σₘ) correlate substitution patterns with reaction rates in SNAr mechanisms .
Basic Question: How can crystallographic data for fluorinated biphenyls inform material design?
Answer:
Single-crystal X-ray diffraction reveals packing motifs (e.g., herringbone vs. layered structures) influenced by fluorine substitution. For example, perfluorinated biphenyls exhibit closer fluorine-fluorine contacts (3.0–3.2 Å), enhancing thermal conductivity in liquid crystal applications .
Properties
CAS No. |
61908-07-4 |
---|---|
Molecular Formula |
C12HF9 |
Molecular Weight |
316.12 g/mol |
IUPAC Name |
1,2,3,4,5-pentafluoro-6-(2,3,4,6-tetrafluorophenyl)benzene |
InChI |
InChI=1S/C12HF9/c13-2-1-3(14)6(15)7(16)4(2)5-8(17)10(19)12(21)11(20)9(5)18/h1H |
InChI Key |
JSMOXNUKSCAGGD-UHFFFAOYSA-N |
Canonical SMILES |
C1=C(C(=C(C(=C1F)F)F)C2=C(C(=C(C(=C2F)F)F)F)F)F |
Origin of Product |
United States |
Disclaimer and Information on In-Vitro Research Products
Please be aware that all articles and product information presented on BenchChem are intended solely for informational purposes. The products available for purchase on BenchChem are specifically designed for in-vitro studies, which are conducted outside of living organisms. In-vitro studies, derived from the Latin term "in glass," involve experiments performed in controlled laboratory settings using cells or tissues. It is important to note that these products are not categorized as medicines or drugs, and they have not received approval from the FDA for the prevention, treatment, or cure of any medical condition, ailment, or disease. We must emphasize that any form of bodily introduction of these products into humans or animals is strictly prohibited by law. It is essential to adhere to these guidelines to ensure compliance with legal and ethical standards in research and experimentation.