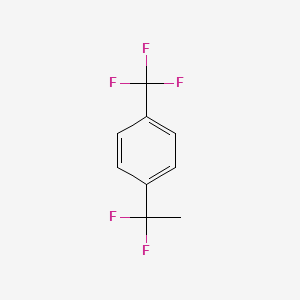
1-(1,1-Difluoroethyl)-4-(trifluoromethyl)benzene
Overview
Description
1-(1,1-Difluoroethyl)-4-(trifluoromethyl)benzene is a useful research compound. Its molecular formula is C9H7F5 and its molecular weight is 210.14 g/mol. The purity is usually 95%.
BenchChem offers high-quality this compound suitable for many research applications. Different packaging options are available to accommodate customers' requirements. Please inquire for more information about this compound including the price, delivery time, and more detailed information at info@benchchem.com.
Mechanism of Action
Target of Action
Compounds with trifluoromethyl groups are known to play an increasingly important role in pharmaceuticals, agrochemicals, and materials .
Mode of Action
It’s known that trifluoromethylation involves the addition of a trifluoromethyl group to carbon-centered radical intermediates .
Biochemical Pathways
The trifluoromethylation process, which this compound may be involved in, is a key transformation in the research and development of drugs, agrochemicals, and functional materials .
Result of Action
The introduction of a trifluoromethyl group onto aromatic rings is a significant transformation in medicinal chemistry and related fields .
Biochemical Analysis
Biochemical Properties
1-(1,1-Difluoroethyl)-4-(trifluoromethyl)benzene plays a crucial role in various biochemical reactions due to its ability to interact with enzymes, proteins, and other biomolecules. The trifluoromethyl group, in particular, is known to enhance the lipophilicity and metabolic stability of the compound, which can influence its interaction with biological targets . This compound has been shown to interact with cytochrome P450 enzymes, which are involved in the metabolism of many drugs and xenobiotics . The nature of these interactions often involves the inhibition or modulation of enzyme activity, leading to altered metabolic pathways.
Cellular Effects
The effects of this compound on various cell types and cellular processes are profound. This compound can influence cell function by modulating cell signaling pathways, gene expression, and cellular metabolism . For instance, it has been observed to affect the expression of genes involved in oxidative stress response and apoptosis. Additionally, this compound can alter cellular metabolism by affecting the activity of key metabolic enzymes, leading to changes in energy production and utilization.
Molecular Mechanism
At the molecular level, this compound exerts its effects through various mechanisms. One of the primary mechanisms involves the binding interactions with biomolecules, such as enzymes and receptors . This compound can act as an enzyme inhibitor or activator, depending on the specific target. For example, it has been shown to inhibit the activity of certain cytochrome P450 enzymes, leading to reduced metabolism of specific substrates . Additionally, this compound can influence gene expression by modulating transcription factors and other regulatory proteins.
Temporal Effects in Laboratory Settings
The temporal effects of this compound in laboratory settings are influenced by its stability, degradation, and long-term effects on cellular function. Studies have shown that this compound is relatively stable under standard laboratory conditions, with minimal degradation over time . Prolonged exposure to this compound can lead to cumulative effects on cellular function, including changes in gene expression and metabolic activity.
Dosage Effects in Animal Models
The effects of this compound vary with different dosages in animal models. At low doses, this compound may exhibit minimal or no adverse effects, while higher doses can lead to toxicity and other adverse effects . Threshold effects have been observed, where a certain dosage level must be reached before significant biochemical or physiological changes occur. High doses of this compound can result in hepatotoxicity, nephrotoxicity, and other organ-specific toxicities.
Metabolic Pathways
This compound is involved in several metabolic pathways, primarily mediated by cytochrome P450 enzymes . These enzymes catalyze the oxidation of the compound, leading to the formation of various metabolites. The interaction with these enzymes can affect metabolic flux and alter the levels of specific metabolites. Additionally, this compound can influence the activity of other metabolic enzymes, further impacting metabolic pathways.
Transport and Distribution
The transport and distribution of this compound within cells and tissues are facilitated by specific transporters and binding proteins . This compound can be actively transported across cell membranes and distributed to various cellular compartments. The localization and accumulation of this compound can influence its biochemical activity and interactions with target biomolecules.
Subcellular Localization
The subcellular localization of this compound is critical for its activity and function. This compound can be directed to specific cellular compartments or organelles through targeting signals or post-translational modifications . The localization within subcellular structures can affect its interactions with enzymes, receptors, and other biomolecules, thereby modulating its biochemical effects.
Biological Activity
Overview
1-(1,1-Difluoroethyl)-4-(trifluoromethyl)benzene, also known as difluoroethyltrifluorobenzene, is a fluorinated aromatic compound that has garnered interest in various scientific fields due to its unique chemical properties and potential biological activities. This article delves into the biological activity of this compound, synthesizing findings from diverse research studies.
Chemical Structure and Properties
The chemical structure of this compound includes a benzene ring substituted with a trifluoromethyl group and a difluoroethyl group. The presence of multiple fluorine atoms significantly influences its physicochemical properties, including lipophilicity and stability.
Antimicrobial Properties
Research indicates that compounds with trifluoromethyl groups often exhibit enhanced biological activities, particularly against bacterial strains. For instance, studies have shown that similar compounds demonstrate significant antimicrobial effects against Staphylococcus aureus and methicillin-resistant Staphylococcus aureus (MRSA) isolates. The minimum inhibitory concentrations (MICs) for these compounds were reported to be as low as 12.9 μM, suggesting potent antibacterial activity .
The biological activity of this compound is hypothesized to involve interactions with specific molecular targets such as enzymes or receptors. The trifluoromethyl group may enhance the compound's binding affinity to these targets due to its electron-withdrawing properties, which can stabilize interactions with nucleophilic sites on proteins .
Toxicological Studies
Toxicological evaluations have been conducted to assess the safety profile of this compound. In animal studies, exposure to high doses resulted in observable adverse effects such as hepatocellular hypertrophy and nephropathy at doses starting from 50 mg/kg body weight . The no-observed-adverse-effect level (NOAEL) was determined to be 10 mg/kg based on liver and kidney effects.
Table: Summary of Biological Activities
Properties
IUPAC Name |
1-(1,1-difluoroethyl)-4-(trifluoromethyl)benzene | |
---|---|---|
Source | PubChem | |
URL | https://pubchem.ncbi.nlm.nih.gov | |
Description | Data deposited in or computed by PubChem | |
InChI |
InChI=1S/C9H7F5/c1-8(10,11)6-2-4-7(5-3-6)9(12,13)14/h2-5H,1H3 | |
Source | PubChem | |
URL | https://pubchem.ncbi.nlm.nih.gov | |
Description | Data deposited in or computed by PubChem | |
InChI Key |
NMDOQCHYHYSMOF-UHFFFAOYSA-N | |
Source | PubChem | |
URL | https://pubchem.ncbi.nlm.nih.gov | |
Description | Data deposited in or computed by PubChem | |
Canonical SMILES |
CC(C1=CC=C(C=C1)C(F)(F)F)(F)F | |
Source | PubChem | |
URL | https://pubchem.ncbi.nlm.nih.gov | |
Description | Data deposited in or computed by PubChem | |
Molecular Formula |
C9H7F5 | |
Source | PubChem | |
URL | https://pubchem.ncbi.nlm.nih.gov | |
Description | Data deposited in or computed by PubChem | |
Molecular Weight |
210.14 g/mol | |
Source | PubChem | |
URL | https://pubchem.ncbi.nlm.nih.gov | |
Description | Data deposited in or computed by PubChem | |
Retrosynthesis Analysis
AI-Powered Synthesis Planning: Our tool employs the Template_relevance Pistachio, Template_relevance Bkms_metabolic, Template_relevance Pistachio_ringbreaker, Template_relevance Reaxys, Template_relevance Reaxys_biocatalysis model, leveraging a vast database of chemical reactions to predict feasible synthetic routes.
One-Step Synthesis Focus: Specifically designed for one-step synthesis, it provides concise and direct routes for your target compounds, streamlining the synthesis process.
Accurate Predictions: Utilizing the extensive PISTACHIO, BKMS_METABOLIC, PISTACHIO_RINGBREAKER, REAXYS, REAXYS_BIOCATALYSIS database, our tool offers high-accuracy predictions, reflecting the latest in chemical research and data.
Strategy Settings
Precursor scoring | Relevance Heuristic |
---|---|
Min. plausibility | 0.01 |
Model | Template_relevance |
Template Set | Pistachio/Bkms_metabolic/Pistachio_ringbreaker/Reaxys/Reaxys_biocatalysis |
Top-N result to add to graph | 6 |
Feasible Synthetic Routes
Disclaimer and Information on In-Vitro Research Products
Please be aware that all articles and product information presented on BenchChem are intended solely for informational purposes. The products available for purchase on BenchChem are specifically designed for in-vitro studies, which are conducted outside of living organisms. In-vitro studies, derived from the Latin term "in glass," involve experiments performed in controlled laboratory settings using cells or tissues. It is important to note that these products are not categorized as medicines or drugs, and they have not received approval from the FDA for the prevention, treatment, or cure of any medical condition, ailment, or disease. We must emphasize that any form of bodily introduction of these products into humans or animals is strictly prohibited by law. It is essential to adhere to these guidelines to ensure compliance with legal and ethical standards in research and experimentation.