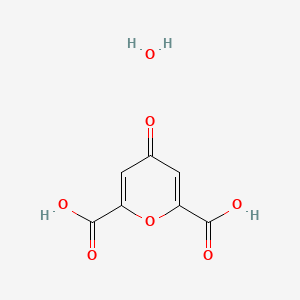
Chelidonic acid monohydrate
Overview
Description
Chelidonic acid monohydrate (CAS 6003-94-7) is a γ-pyrone derivative with the molecular formula C₇H₆O₇·H₂O. It is a dicarboxylic acid featuring two carboxylic acid groups at the 2- and 6-positions of the 4-oxo-4H-pyran ring . Naturally occurring in plants such as Chelidonium majus (Papaveraceae) and hyperaccumulators like Berkheya coddii, it plays roles in metal ion chelation and stress response . Its biological activities include anti-inflammatory, antioxidant, and osteogenic properties, with demonstrated effects in downregulating senescence markers (e.g., p16) and modulating interleukin (IL)-1β, IL-6, and TNF-α pathways .
Structurally, this compound forms hydrogen-bonded networks in its crystalline methanol solvate, characterized by elongated C=O bonds (1.240 Å experimentally vs. 1.219 Å theoretically) due to intermolecular H-bonding . It exhibits C₂ symmetry and serves as a precursor for synthesizing γ-pyrone derivatives, such as 4H-pyran-4-one, via decarboxylation .
Preparation Methods
Chelidonic acid can be synthesized through a two-step process involving diethyl oxalate and acetone . The reaction conditions typically involve the use of a base to facilitate the formation of the pyrone ring structure. Industrial production methods may vary, but they generally follow similar synthetic routes with optimizations for yield and purity.
Chemical Reactions Analysis
Chelidonic acid monohydrate undergoes several types of chemical reactions, including:
Oxidation: Chelidonic acid can be oxidized to form various oxidized derivatives.
Reduction: Reduction reactions can convert chelidonic acid into its reduced forms.
Substitution: Substitution reactions often involve the replacement of hydrogen atoms with other functional groups. Common reagents used in these reactions include oxidizing agents like potassium permanganate and reducing agents like sodium borohydride.
Scientific Research Applications
Pharmacological Applications
Chelidonic acid and its derivatives demonstrate significant pharmacological potential. The following subsections detail its various therapeutic uses.
Analgesic and Anti-inflammatory Properties
Research indicates that chelidonic acid possesses mild analgesic and anti-inflammatory effects. It has been shown to inhibit the production of pro-inflammatory cytokines such as IL-6, suggesting its potential in treating inflammatory diseases .
- Case Study : A study on the effects of chelidonic acid on mast cell degranulation in rats revealed a decrease in allergic responses, indicating its utility in managing allergic conditions .
Antimicrobial Activity
Chelidonic acid exhibits antimicrobial properties against various pathogens. It has been investigated for its effectiveness against bacterial strains and fungi, making it a candidate for developing new antimicrobial agents .
- Case Study : In vitro experiments demonstrated that chelidonic acid could inhibit the growth of specific pathogenic bacteria, supporting its application in infection control .
Osteogenic Activity
The compound is recognized for its osteogenic properties, particularly when complexed with calcium to form calcium chelidonate. This complex stimulates the viability and differentiation of mesenchymal stem cells, enhancing bone tissue regeneration .
- Case Study : In vivo studies showed that calcium chelidonate significantly improved bone healing in animal models, suggesting its potential for use in bone tissue engineering and regenerative medicine .
Chemical and Material Science Applications
Beyond its biological applications, chelidonic acid monohydrate is valuable in material science.
Chelating Agent
Chelidonic acid acts as an effective chelating agent for various metal ions, which is crucial in fields such as environmental science and analytical chemistry. Its ability to form stable complexes with metals enhances its utility in remediation technologies and catalysis .
- Data Table: Chelating Properties of this compound
Metal Ion | Chelation Strength | Application Area |
---|---|---|
Calcium | High | Bone regeneration |
Iron | Moderate | Environmental remediation |
Copper | Low | Catalytic processes |
Synthesis of Organometallic Complexes
Chelidonic acid has been utilized to synthesize various organometallic complexes, which are of interest for their unique electronic properties and potential applications in catalysis and drug delivery systems .
Mechanism of Action
The mechanism of action of chelidonic acid involves its interaction with various molecular targets and pathways. It has been shown to inhibit enzymes like glutamate decarboxylase, which plays a role in neurotransmitter regulation . Additionally, chelidonic acid can modulate oxidative stress pathways, thereby exerting its antioxidant effects .
Comparison with Similar Compounds
Structural Analogs
2.1.1. Isophthalic Acid
- Structure : A benzene derivative with two carboxylic acid groups at the 1,3-positions.
- Key Differences: Lacks the γ-pyrone ring, resulting in distinct electronic properties and aromaticity indices. Forms typical carboxylic acid dimers via strong H-bonds (e.g., in formic acid-like motifs), unlike chelidonic acid’s methanol-solvated H-bond network .
2.1.2. Comanic Acid (4-Oxo-4H-pyran-2-carboxylic Acid; CAS 499-05-8)
- Structure: A monocarboxylic γ-pyrone derivative.
- Key Differences: Reduced acidity and chelation capacity due to a single carboxylic group. Limited bioactivity data compared to chelidonic acid’s well-documented roles in cellular senescence and metal hyperaccumulation .
Derivatives and Salts
2.2.1. Methyl Monoester of Chelidonic Acid
- Structure : One carboxylic acid group esterified to methyl.
- Key Differences :
2.2.2. Saucalchelin (Calcium Complex of Chelidonic Acid)
- Structure : [Ca(ChA)(H₂O)₃], a calcium-chelate complex.
- Key Differences :
2.2.3. Potassium Chelidonate (CAS 108490-88-6)
- Structure : Potassium salt of chelidonic acid.
- Key Differences :
Functional Analogs
2.3.1. Glyoxylic Acid Monohydrate (CAS 563-96-2)
- Structure : A linear α-keto acid (HOOC–CHO·H₂O).
- Key Differences: Lacks aromaticity, leading to divergent reactivity (e.g., used in vanillin synthesis vs. chelidonic acid’s role in heterocyclic chemistry) . No reported anti-inflammatory or metal-chelating activity.
Comparative Data Tables
Table 1: Structural and Physicochemical Properties
Research Implications
This compound’s unique γ-pyrone scaffold and dicarboxylic functionality distinguish it from linear analogs (e.g., glyoxylic acid) and aromatic derivatives (e.g., isophthalic acid). Its calcium complex, saucalchelin, demonstrates the importance of metal coordination in enhancing bioactivity, particularly in osteogenesis . Future studies should explore structure-activity relationships (SAR) of esterified derivatives and salts for targeted drug delivery, leveraging its dual anti-inflammatory and metal-chelating properties .
Biological Activity
Chelidonic acid monohydrate, a derivative of the plant Chelidonium majus, has garnered attention due to its diverse biological activities. This article explores its pharmacological effects, mechanisms of action, and potential therapeutic applications, supported by case studies and research findings.
Overview of Chelidonic Acid
Chelidonic acid is a pyrone compound that exhibits various biological activities, including analgesic, antimicrobial, anti-inflammatory, and oncostatic effects. It is primarily derived from medicinal plants and has been studied for its potential in treating conditions such as allergies, diabetes, and inflammatory diseases .
1. Analgesic and Anti-inflammatory Effects
Chelidonic acid has demonstrated mild analgesic properties. In a study involving a model of allergic rhinitis, it was shown to inhibit mast cell degranulation, suggesting a role in modulating immune responses . Additionally, it has been implicated in reducing inflammation through various pathways.
2. Osteogenic Properties
Despite its low native osteogenic activity, chelidonic acid can form complexes with minerals like calcium, enhancing its osteogenic potential. Studies indicate that calcium chelidonate significantly stimulates mesenchymal stem cell viability and promotes osteogenic differentiation, making it a candidate for bone tissue engineering .
3. Antimicrobial Activity
Research indicates that chelidonic acid possesses antimicrobial properties. It has been effective against certain bacterial strains and fungi, contributing to its potential as an alternative therapeutic agent in infectious diseases .
Chelidonic acid's biological effects are mediated through several mechanisms:
- Inhibition of Enzymatic Activity : Chelidonic acid acts as an inhibitor of glutamate decarboxylase in the rat brain, which may contribute to its analgesic effects .
- Molecular Interactions : The ability to form complexes with metal ions enhances its stability and bioavailability, facilitating targeted delivery of therapeutic agents within the body .
- Regulation of Immune Responses : By modulating mast cell activity and cytokine production, chelidonic acid may help alleviate allergic reactions and inflammatory responses .
Case Study 1: Allergic Rhinitis
In a study on allergic rhinitis models, chelidonic acid was administered to evaluate its effects on symptoms and immune response. The results indicated a significant reduction in nasal inflammation and improved respiratory function compared to control groups .
Case Study 2: Osteogenic Activity
A series of experiments were conducted using calcium chelidonate on mesenchymal stem cells. The findings revealed enhanced cell adhesion and mineralization of the extracellular matrix, supporting its application in bone regeneration therapies .
Future Directions
The potential applications of this compound extend into various fields such as pharmacology and regenerative medicine. Future research should focus on:
- Clinical Trials : Conducting human trials to validate the efficacy and safety of chelidonic acid in treating specific conditions.
- Mechanistic Studies : Further elucidating the molecular pathways through which chelidonic acid exerts its biological effects.
- Formulation Development : Exploring novel delivery systems that enhance the bioavailability and therapeutic outcomes of chelidonic acid derivatives.
Q & A
Basic Research Questions
Q. How is the crystal structure of chelidonic acid monohydrate characterized experimentally?
The crystal structure of this compound is determined using X-ray crystallography. For example, the methanol solvate structure reveals hydrogen bonding networks (e.g., two short H-bonds: 1.685 Å and 2.142 Å) and solvent interactions, with bond lengths such as the carbonyl (C=O) bond at 1.240 Å experimentally versus 1.219 Å in gas-phase DFT calculations . These discrepancies arise from intermolecular interactions in the crystal state, which are absent in isolated molecule simulations .
Q. What methodological considerations are critical for preparing chelidonic acid solutions in laboratory settings?
Chelidonic acid is supplied as a crystalline solid with limited aqueous solubility. For biological assays, dissolve it in DMSO (1 mg/mL) and dilute with buffered solutions (e.g., PBS pH 7.2) to achieve ~0.14 mg/mL. Avoid prolonged storage of aqueous solutions (>24 hours) due to instability .
Q. What are the primary pharmacological mechanisms of chelidonic acid in inflammation models?
Chelidonic acid inhibits NF-κB and caspase-1 pathways, reducing IL-6 and TNF-α production in murine colitis models. It also lowers COX-2 and PGE2 levels in the colon, demonstrating dose-dependent anti-inflammatory effects .
Advanced Research Questions
Q. How do solvent effects and theoretical modeling (e.g., DFT-B3LYP) influence structural predictions of chelidonic acid?
Gas-phase DFT-B3LYP/CBS(T-5) calculations predict bond lengths (e.g., C=O at 1.219 Å) that differ from experimental X-ray data (1.240 Å) due to hydrogen bonding in the crystal state. Polar solvents further elongate bonds by ~0.04 Å, highlighting the need to account for solvent interactions in computational models .
Q. How can researchers resolve contradictions between theoretical and experimental dipole moments in chelidonic acid?
Theoretical dipole moments (calculated via B3LYP/aug-cc-pVXZ) may deviate from experimental values due to solvent polarity and crystal packing. For example, polar solvents increase dipole moments by stabilizing charge separation, necessitating hybrid QM/MM approaches to model solvation effects accurately .
Q. What experimental design is optimal for studying chelidonic acid’s dose-dependent effects on cellular senescence?
Use oxidative stress-induced models (e.g., H₂O₂-treated human fibroblasts) with chelidonic acid concentrations (250–500 µM). Quantify senescence markers like p16 protein levels via Western blot. Significant reductions in p16 (p < 0.0001 at 500 µM) confirm dose-dependent efficacy .
Q. How does chelidonic acid modulate glutamic acid decarboxylase (GAD) activity, and what are the implications for neurological research?
Chelidonic acid acts as a competitive GAD inhibitor (Ki = 1.2 µM) by binding to the active site, reducing GABA synthesis. This mechanism is critical for studying neurotransmitter regulation in conditions like epilepsy or anxiety. Validate inhibition via enzymatic assays with ¹³C-labeled substrates .
Q. What strategies mitigate discrepancies in chelidonic acid’s spectroscopic data (IR, Raman) between theoretical and experimental results?
Combine DFT-predicted vibrational frequencies with experimental IR/Raman spectra, adjusting for solvent and crystal lattice effects. For example, H-bonding in the chelidonic acid–methanol dimer shifts O-H stretching frequencies, requiring explicit solvent modeling in simulations .
Q. Methodological Guidance
Q. How to analyze chelidonic acid’s H-bonding network in crystal structures?
Use Cambridge Structural Database (CSD) searches to compare H-bond motifs with related carboxylic acids (e.g., isophthalic acid). Calculate interaction energies (e.g., −13.22 kcal/mol for methanol dimer) via DFT to quantify stability .
Q. What computational tools validate chelidonic acid’s aromaticity indices (HOMA, NICS)?
Apply B3LYP/CBS methods to compute geometric (HOMA) and magnetic (NICS) aromaticity indices. For chelidonic acid, HOMA values <0.5 indicate reduced aromaticity due to electron-withdrawing carboxyl groups, aligning with NICS(1) values from GIAO calculations .
Q. Data Contradictions and Resolution
Q. Why do theoretical models underestimate chelidonic acid’s bioactivity in hydrophobic environments?
Most DFT studies assume aqueous or gas-phase conditions, neglecting lipid bilayer interactions. Use molecular dynamics (MD) simulations with membrane-embedded models to assess partitioning and binding to hydrophobic targets (e.g., COX-2) .
Q. How to reconcile conflicting reports on chelidonic acid’s role in IgE modulation?
In murine allergy models, chelidonic acid increases serum IgE but reduces splenic IFN-γ. Context-dependent effects (e.g., tissue-specific NF-κB activation) require multiplex cytokine profiling and single-cell RNA-seq to delineate pathways .
Properties
IUPAC Name |
4-oxopyran-2,6-dicarboxylic acid;hydrate | |
---|---|---|
Source | PubChem | |
URL | https://pubchem.ncbi.nlm.nih.gov | |
Description | Data deposited in or computed by PubChem | |
InChI |
InChI=1S/C7H4O6.H2O/c8-3-1-4(6(9)10)13-5(2-3)7(11)12;/h1-2H,(H,9,10)(H,11,12);1H2 | |
Source | PubChem | |
URL | https://pubchem.ncbi.nlm.nih.gov | |
Description | Data deposited in or computed by PubChem | |
InChI Key |
KJZJAKSEIQOKAK-UHFFFAOYSA-N | |
Source | PubChem | |
URL | https://pubchem.ncbi.nlm.nih.gov | |
Description | Data deposited in or computed by PubChem | |
Canonical SMILES |
C1=C(OC(=CC1=O)C(=O)O)C(=O)O.O | |
Source | PubChem | |
URL | https://pubchem.ncbi.nlm.nih.gov | |
Description | Data deposited in or computed by PubChem | |
Molecular Formula |
C7H6O7 | |
Source | PubChem | |
URL | https://pubchem.ncbi.nlm.nih.gov | |
Description | Data deposited in or computed by PubChem | |
Molecular Weight |
202.12 g/mol | |
Source | PubChem | |
URL | https://pubchem.ncbi.nlm.nih.gov | |
Description | Data deposited in or computed by PubChem | |
CAS No. |
6003-94-7 | |
Record name | Chelidonic Acid Monohydrate | |
Source | European Chemicals Agency (ECHA) | |
URL | https://echa.europa.eu/information-on-chemicals | |
Description | The European Chemicals Agency (ECHA) is an agency of the European Union which is the driving force among regulatory authorities in implementing the EU's groundbreaking chemicals legislation for the benefit of human health and the environment as well as for innovation and competitiveness. | |
Explanation | Use of the information, documents and data from the ECHA website is subject to the terms and conditions of this Legal Notice, and subject to other binding limitations provided for under applicable law, the information, documents and data made available on the ECHA website may be reproduced, distributed and/or used, totally or in part, for non-commercial purposes provided that ECHA is acknowledged as the source: "Source: European Chemicals Agency, http://echa.europa.eu/". Such acknowledgement must be included in each copy of the material. ECHA permits and encourages organisations and individuals to create links to the ECHA website under the following cumulative conditions: Links can only be made to webpages that provide a link to the Legal Notice page. | |
Retrosynthesis Analysis
AI-Powered Synthesis Planning: Our tool employs the Template_relevance Pistachio, Template_relevance Bkms_metabolic, Template_relevance Pistachio_ringbreaker, Template_relevance Reaxys, Template_relevance Reaxys_biocatalysis model, leveraging a vast database of chemical reactions to predict feasible synthetic routes.
One-Step Synthesis Focus: Specifically designed for one-step synthesis, it provides concise and direct routes for your target compounds, streamlining the synthesis process.
Accurate Predictions: Utilizing the extensive PISTACHIO, BKMS_METABOLIC, PISTACHIO_RINGBREAKER, REAXYS, REAXYS_BIOCATALYSIS database, our tool offers high-accuracy predictions, reflecting the latest in chemical research and data.
Strategy Settings
Precursor scoring | Relevance Heuristic |
---|---|
Min. plausibility | 0.01 |
Model | Template_relevance |
Template Set | Pistachio/Bkms_metabolic/Pistachio_ringbreaker/Reaxys/Reaxys_biocatalysis |
Top-N result to add to graph | 6 |
Feasible Synthetic Routes
Disclaimer and Information on In-Vitro Research Products
Please be aware that all articles and product information presented on BenchChem are intended solely for informational purposes. The products available for purchase on BenchChem are specifically designed for in-vitro studies, which are conducted outside of living organisms. In-vitro studies, derived from the Latin term "in glass," involve experiments performed in controlled laboratory settings using cells or tissues. It is important to note that these products are not categorized as medicines or drugs, and they have not received approval from the FDA for the prevention, treatment, or cure of any medical condition, ailment, or disease. We must emphasize that any form of bodily introduction of these products into humans or animals is strictly prohibited by law. It is essential to adhere to these guidelines to ensure compliance with legal and ethical standards in research and experimentation.