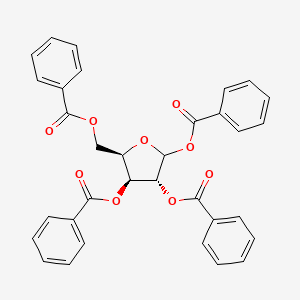
Tetra-O-benzoyl-D-xylofuranose
Overview
Description
Mechanism of Action
Target of Action
1,2,3,4-Tetra-O-benzoyl-D-xylofuranose is primarily used as a reagent in various chemical reactions that involve carbohydrate derivatives . It is a synthetic compound that finds its primary utility in proteomics research .
Mode of Action
It is known to be involved in various chemical reactions that involve carbohydrate derivatives . It is proposed that upon deacetylation, the oxonium intermediate is formed, and the attack of a water molecule on the oxonium intermediate will lead to the formation of the corresponding 2,3,4,6-tetra-O-acetyl α- or β-glucopyranose .
Biochemical Pathways
It is known to be involved in various chemical reactions that involve carbohydrate derivatives .
Biochemical Analysis
Biochemical Properties
1,2,3,4-Tetra-O-benzoyl-D-xylofuranose plays a significant role in biochemical reactions, particularly in the synthesis of complex carbohydrates and glycosides. The compound interacts with various enzymes, such as glycosyltransferases, which facilitate the transfer of glycosyl groups to acceptor molecules. These interactions are crucial for the formation of glycosidic bonds, which are essential for the structure and function of many biomolecules. Additionally, 1,2,3,4-Tetra-O-benzoyl-D-xylofuranose can act as a substrate for esterases, enzymes that hydrolyze ester bonds, leading to the release of benzoyl groups and the formation of free xylofuranose .
Cellular Effects
The effects of 1,2,3,4-Tetra-O-benzoyl-D-xylofuranose on various cell types and cellular processes are diverse. This compound can influence cell function by modulating cell signaling pathways, gene expression, and cellular metabolism. For instance, the presence of benzoyl groups can affect the compound’s ability to interact with cellular receptors and enzymes, thereby altering signal transduction pathways. Additionally, 1,2,3,4-Tetra-O-benzoyl-D-xylofuranose may impact gene expression by acting as a chemical modifier of DNA or histones, leading to changes in chromatin structure and transcriptional activity .
Molecular Mechanism
At the molecular level, 1,2,3,4-Tetra-O-benzoyl-D-xylofuranose exerts its effects through various binding interactions with biomolecules. The benzoyl groups on the compound can form non-covalent interactions, such as hydrogen bonds and hydrophobic interactions, with proteins and nucleic acids. These interactions can influence the activity of enzymes, either by inhibiting or activating them, depending on the specific context. For example, the compound may inhibit glycosidases by binding to their active sites, preventing the hydrolysis of glycosidic bonds .
Temporal Effects in Laboratory Settings
In laboratory settings, the effects of 1,2,3,4-Tetra-O-benzoyl-D-xylofuranose can change over time due to its stability and degradation. The compound is generally stable under standard storage conditions, but it may undergo hydrolysis in the presence of water or acidic conditions, leading to the release of benzoyl groups and the formation of free xylofuranose. Long-term exposure to the compound in in vitro or in vivo studies may result in cumulative effects on cellular function, such as alterations in gene expression and metabolic activity .
Dosage Effects in Animal Models
The effects of 1,2,3,4-Tetra-O-benzoyl-D-xylofuranose in animal models can vary with different dosages. At low doses, the compound may exhibit minimal effects on cellular function and overall health. At higher doses, it may cause toxic or adverse effects, such as cellular stress, apoptosis, or organ damage. Threshold effects may also be observed, where a specific dosage level triggers a significant change in biological activity .
Metabolic Pathways
1,2,3,4-Tetra-O-benzoyl-D-xylofuranose is involved in various metabolic pathways, particularly those related to carbohydrate metabolism. The compound can be metabolized by esterases, leading to the release of benzoyl groups and the formation of free xylofuranose. This free xylofuranose can then enter glycolytic or pentose phosphate pathways, contributing to energy production and biosynthesis of nucleotides and amino acids .
Transport and Distribution
Within cells and tissues, 1,2,3,4-Tetra-O-benzoyl-D-xylofuranose is transported and distributed through various mechanisms. The compound may interact with specific transporters or binding proteins that facilitate its uptake and localization. Additionally, the benzoyl groups on the compound can influence its solubility and membrane permeability, affecting its distribution within different cellular compartments .
Subcellular Localization
The subcellular localization of 1,2,3,4-Tetra-O-benzoyl-D-xylofuranose can impact its activity and function. The compound may be directed to specific compartments or organelles through targeting signals or post-translational modifications. For example, the presence of benzoyl groups may facilitate the compound’s localization to the endoplasmic reticulum or Golgi apparatus, where it can participate in glycosylation reactions .
Preparation Methods
Tetra-O-benzoyl-D-xylofuranose can be synthesized through several synthetic routes. One common method involves the benzoylation of D-xylofuranose. The reaction typically uses benzoyl chloride in the presence of a base such as pyridine. The reaction conditions often require a controlled temperature and an inert atmosphere to prevent side reactions. Industrial production methods may involve similar steps but are scaled up and optimized for higher yields and purity.
Chemical Reactions Analysis
Tetra-O-benzoyl-D-xylofuranose undergoes various chemical reactions, including:
Oxidation: This compound can be oxidized to form different derivatives, depending on the reagents and conditions used.
Reduction: Reduction reactions can convert it into simpler carbohydrate derivatives.
Substitution: It can undergo substitution reactions where the benzoyl groups are replaced with other functional groups.
Common reagents used in these reactions include oxidizing agents like potassium permanganate and reducing agents like sodium borohydride. The major products formed from these reactions depend on the specific conditions and reagents used .
Scientific Research Applications
Tetra-O-benzoyl-D-xylofuranose has a wide range of scientific research applications:
Chemistry: It is used as a building block in the synthesis of more complex carbohydrate molecules and nucleosides.
Biology: Researchers use it to study carbohydrate metabolism and enzyme interactions.
Industry: It is used in the production of various carbohydrate-based products and materials.
Comparison with Similar Compounds
Tetra-O-benzoyl-D-xylofuranose can be compared with other similar compounds such as:
1,2,3,4-Tetra-O-acetyl-D-glucopyranose: Another carbohydrate derivative used in similar synthetic applications.
2,3,4,6-Tetra-O-benzoyl-D-glucopyranose: A compound with similar benzoyl groups but different sugar backbone.
1,2,3,4-Tetra-O-benzoyl-L-fucopyranose: A similar compound with a different stereochemistry.
The uniqueness of this compound lies in its specific structure and reactivity, making it particularly useful in the synthesis of certain nucleosides and carbohydrate-based molecules .
Properties
IUPAC Name |
(3,4,5-tribenzoyloxyoxolan-2-yl)methyl benzoate | |
---|---|---|
Details | Computed by Lexichem TK 2.7.0 (PubChem release 2021.05.07) | |
Source | PubChem | |
URL | https://pubchem.ncbi.nlm.nih.gov | |
Description | Data deposited in or computed by PubChem | |
InChI |
InChI=1S/C33H26O9/c34-29(22-13-5-1-6-14-22)38-21-26-27(40-30(35)23-15-7-2-8-16-23)28(41-31(36)24-17-9-3-10-18-24)33(39-26)42-32(37)25-19-11-4-12-20-25/h1-20,26-28,33H,21H2 | |
Details | Computed by InChI 1.0.6 (PubChem release 2021.05.07) | |
Source | PubChem | |
URL | https://pubchem.ncbi.nlm.nih.gov | |
Description | Data deposited in or computed by PubChem | |
InChI Key |
SVVKRZQAFRVDED-UHFFFAOYSA-N | |
Details | Computed by InChI 1.0.6 (PubChem release 2021.05.07) | |
Source | PubChem | |
URL | https://pubchem.ncbi.nlm.nih.gov | |
Description | Data deposited in or computed by PubChem | |
Canonical SMILES |
C1=CC=C(C=C1)C(=O)OCC2C(C(C(O2)OC(=O)C3=CC=CC=C3)OC(=O)C4=CC=CC=C4)OC(=O)C5=CC=CC=C5 | |
Details | Computed by OEChem 2.3.0 (PubChem release 2021.05.07) | |
Source | PubChem | |
URL | https://pubchem.ncbi.nlm.nih.gov | |
Description | Data deposited in or computed by PubChem | |
Molecular Formula |
C33H26O9 | |
Details | Computed by PubChem 2.1 (PubChem release 2021.05.07) | |
Source | PubChem | |
URL | https://pubchem.ncbi.nlm.nih.gov | |
Description | Data deposited in or computed by PubChem | |
Molecular Weight |
566.6 g/mol | |
Details | Computed by PubChem 2.1 (PubChem release 2021.05.07) | |
Source | PubChem | |
URL | https://pubchem.ncbi.nlm.nih.gov | |
Description | Data deposited in or computed by PubChem | |
CAS No. |
5432-87-1 | |
Record name | NSC25067 | |
Source | DTP/NCI | |
URL | https://dtp.cancer.gov/dtpstandard/servlet/dwindex?searchtype=NSC&outputformat=html&searchlist=25067 | |
Description | The NCI Development Therapeutics Program (DTP) provides services and resources to the academic and private-sector research communities worldwide to facilitate the discovery and development of new cancer therapeutic agents. | |
Explanation | Unless otherwise indicated, all text within NCI products is free of copyright and may be reused without our permission. Credit the National Cancer Institute as the source. | |
Retrosynthesis Analysis
AI-Powered Synthesis Planning: Our tool employs the Template_relevance Pistachio, Template_relevance Bkms_metabolic, Template_relevance Pistachio_ringbreaker, Template_relevance Reaxys, Template_relevance Reaxys_biocatalysis model, leveraging a vast database of chemical reactions to predict feasible synthetic routes.
One-Step Synthesis Focus: Specifically designed for one-step synthesis, it provides concise and direct routes for your target compounds, streamlining the synthesis process.
Accurate Predictions: Utilizing the extensive PISTACHIO, BKMS_METABOLIC, PISTACHIO_RINGBREAKER, REAXYS, REAXYS_BIOCATALYSIS database, our tool offers high-accuracy predictions, reflecting the latest in chemical research and data.
Strategy Settings
Precursor scoring | Relevance Heuristic |
---|---|
Min. plausibility | 0.01 |
Model | Template_relevance |
Template Set | Pistachio/Bkms_metabolic/Pistachio_ringbreaker/Reaxys/Reaxys_biocatalysis |
Top-N result to add to graph | 6 |
Feasible Synthetic Routes
Disclaimer and Information on In-Vitro Research Products
Please be aware that all articles and product information presented on BenchChem are intended solely for informational purposes. The products available for purchase on BenchChem are specifically designed for in-vitro studies, which are conducted outside of living organisms. In-vitro studies, derived from the Latin term "in glass," involve experiments performed in controlled laboratory settings using cells or tissues. It is important to note that these products are not categorized as medicines or drugs, and they have not received approval from the FDA for the prevention, treatment, or cure of any medical condition, ailment, or disease. We must emphasize that any form of bodily introduction of these products into humans or animals is strictly prohibited by law. It is essential to adhere to these guidelines to ensure compliance with legal and ethical standards in research and experimentation.