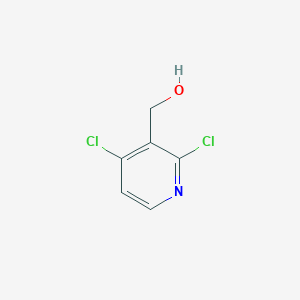
(2,4-Dichloropyridin-3-yl)methanol
Overview
Description
“(2,4-Dichloropyridin-3-yl)methanol” is a chemical compound with the molecular formula C6H5Cl2NO . It has a molecular weight of 178.02 . It is a solid substance that is stored in an inert atmosphere at temperatures between 2-8°C .
Molecular Structure Analysis
The InChI code for “this compound” is 1S/C6H5Cl2NO/c7-5-1-2-9-6(8)4(5)3-10/h1-2,10H,3H2 . This code provides a standard way to encode the compound’s molecular structure.Physical And Chemical Properties Analysis
“this compound” is a solid substance . It is stored in an inert atmosphere at temperatures between 2-8°C .Scientific Research Applications
Biocatalytic Synthesis in Microreaction Systems One significant application of related pyridinyl methanol compounds is in the biocatalytic synthesis processes. For example, the synthesis of S-(4-chlorophenyl)-(pyridin-2-yl) methanol (S-CPMA) utilizes recombinant Escherichia coli in a water-cyclohexane system, showcasing an innovative, green, and efficient method. This approach emphasizes the use of microreaction systems with membrane dispersion and 3D bending microchannels to enhance mass transfer efficiency and reduce synthesis time significantly, achieving yields of 99.6% with high enantiomeric excess in just 80 minutes (Chen et al., 2021).
Microbial Production of Chiral Intermediates Another research application involves the microbial production of key chiral intermediates, such as the production of (S)-CPMA, a critical intermediate for anti-allergic drugs like Betahistine. This process utilizes carbonyl reductase-producing microorganisms isolated from soil, highlighting the potential of biotechnology in synthesizing chiral compounds efficiently and sustainably. The use of an aqueous two-phase system (ATPS) demonstrated improved substrate tolerance and biocompatibility, leading to enhanced yields and enantiomeric excess of the product (Ni et al., 2012).
Catalytic Applications in Polymerization The synthesis of nickel complexes with bidentate N,O-type ligands, including pyridinyl methanol derivatives, showcases their utility in catalysis, particularly in the oligomerization of ethylene. These complexes demonstrate the potential for high-yield polymerization processes, with specific nickel complexes showing notable activity and turnover frequencies. Such research underlines the role of pyridinyl methanol derivatives in developing more efficient and selective catalysts for industrial applications (Kermagoret & Braunstein, 2008).
Hybrid Organic-Inorganic Material Synthesis The creation of hybrid organic-inorganic materials, utilizing pyridinyl methanol derivatives, has been explored for their unique structures and photophysical properties. These materials, synthesized through oxidative condensation-cyclization processes, have potential applications in optoelectronics and catalysis due to their interesting structural characteristics and emission properties. This research area opens up new possibilities for the development of materials with tailored properties for specific applications (Buvaylo et al., 2015).
Safety and Hazards
The compound is labeled with the GHS07 pictogram . The hazard statements associated with it are H315 (Causes skin irritation), H319 (Causes serious eye irritation), and H335 (May cause respiratory irritation) . Precautionary measures include avoiding breathing dust/fume/gas/mist/vapors/spray and washing thoroughly after handling .
properties
IUPAC Name |
(2,4-dichloropyridin-3-yl)methanol | |
---|---|---|
Source | PubChem | |
URL | https://pubchem.ncbi.nlm.nih.gov | |
Description | Data deposited in or computed by PubChem | |
InChI |
InChI=1S/C6H5Cl2NO/c7-5-1-2-9-6(8)4(5)3-10/h1-2,10H,3H2 | |
Source | PubChem | |
URL | https://pubchem.ncbi.nlm.nih.gov | |
Description | Data deposited in or computed by PubChem | |
InChI Key |
OUFKQGCYLJSBMZ-UHFFFAOYSA-N | |
Source | PubChem | |
URL | https://pubchem.ncbi.nlm.nih.gov | |
Description | Data deposited in or computed by PubChem | |
Canonical SMILES |
C1=CN=C(C(=C1Cl)CO)Cl | |
Source | PubChem | |
URL | https://pubchem.ncbi.nlm.nih.gov | |
Description | Data deposited in or computed by PubChem | |
Molecular Formula |
C6H5Cl2NO | |
Source | PubChem | |
URL | https://pubchem.ncbi.nlm.nih.gov | |
Description | Data deposited in or computed by PubChem | |
Molecular Weight |
178.01 g/mol | |
Source | PubChem | |
URL | https://pubchem.ncbi.nlm.nih.gov | |
Description | Data deposited in or computed by PubChem | |
CAS RN |
945543-24-8 | |
Record name | (2,4-dichloropyridin-3-yl)methanol | |
Source | European Chemicals Agency (ECHA) | |
URL | https://echa.europa.eu/information-on-chemicals | |
Description | The European Chemicals Agency (ECHA) is an agency of the European Union which is the driving force among regulatory authorities in implementing the EU's groundbreaking chemicals legislation for the benefit of human health and the environment as well as for innovation and competitiveness. | |
Explanation | Use of the information, documents and data from the ECHA website is subject to the terms and conditions of this Legal Notice, and subject to other binding limitations provided for under applicable law, the information, documents and data made available on the ECHA website may be reproduced, distributed and/or used, totally or in part, for non-commercial purposes provided that ECHA is acknowledged as the source: "Source: European Chemicals Agency, http://echa.europa.eu/". Such acknowledgement must be included in each copy of the material. ECHA permits and encourages organisations and individuals to create links to the ECHA website under the following cumulative conditions: Links can only be made to webpages that provide a link to the Legal Notice page. | |
Synthesis routes and methods I
Procedure details
Synthesis routes and methods II
Procedure details
Retrosynthesis Analysis
AI-Powered Synthesis Planning: Our tool employs the Template_relevance Pistachio, Template_relevance Bkms_metabolic, Template_relevance Pistachio_ringbreaker, Template_relevance Reaxys, Template_relevance Reaxys_biocatalysis model, leveraging a vast database of chemical reactions to predict feasible synthetic routes.
One-Step Synthesis Focus: Specifically designed for one-step synthesis, it provides concise and direct routes for your target compounds, streamlining the synthesis process.
Accurate Predictions: Utilizing the extensive PISTACHIO, BKMS_METABOLIC, PISTACHIO_RINGBREAKER, REAXYS, REAXYS_BIOCATALYSIS database, our tool offers high-accuracy predictions, reflecting the latest in chemical research and data.
Strategy Settings
Precursor scoring | Relevance Heuristic |
---|---|
Min. plausibility | 0.01 |
Model | Template_relevance |
Template Set | Pistachio/Bkms_metabolic/Pistachio_ringbreaker/Reaxys/Reaxys_biocatalysis |
Top-N result to add to graph | 6 |
Feasible Synthetic Routes
Disclaimer and Information on In-Vitro Research Products
Please be aware that all articles and product information presented on BenchChem are intended solely for informational purposes. The products available for purchase on BenchChem are specifically designed for in-vitro studies, which are conducted outside of living organisms. In-vitro studies, derived from the Latin term "in glass," involve experiments performed in controlled laboratory settings using cells or tissues. It is important to note that these products are not categorized as medicines or drugs, and they have not received approval from the FDA for the prevention, treatment, or cure of any medical condition, ailment, or disease. We must emphasize that any form of bodily introduction of these products into humans or animals is strictly prohibited by law. It is essential to adhere to these guidelines to ensure compliance with legal and ethical standards in research and experimentation.