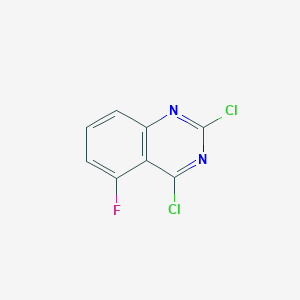
2,4-Dichloro-5-fluoroquinazoline
Overview
Description
2,4-Dichloro-5-fluoroquinazoline is a useful research compound. Its molecular formula is C8H3Cl2FN2 and its molecular weight is 217.02 g/mol. The purity is usually 95%.
BenchChem offers high-quality this compound suitable for many research applications. Different packaging options are available to accommodate customers' requirements. Please inquire for more information about this compound including the price, delivery time, and more detailed information at info@benchchem.com.
Mechanism of Action
The mechanism of action of quinazolines can vary depending on the specific compound and its targets. Generally, they work by interacting with certain enzymes or receptors in the body, leading to changes in biochemical pathways and resulting in various therapeutic effects .
The pharmacokinetics of quinazolines, including their absorption, distribution, metabolism, and excretion (ADME), can also vary widely depending on the specific compound. Factors such as the compound’s chemical structure, formulation, route of administration, and the patient’s physiological condition can all affect its pharmacokinetics .
The action environment, or how environmental factors influence the compound’s action, efficacy, and stability, is another important consideration. Factors such as temperature, pH, and the presence of other substances can affect how a quinazoline compound behaves in the body .
Biochemical Analysis
Biochemical Properties
2,4-Dichloro-5-fluoroquinazoline plays a crucial role in biochemical reactions, particularly in the inhibition of specific enzymes. It interacts with various biomolecules, including proteins and enzymes, through binding interactions that can alter their activity. For instance, this compound has been shown to inhibit certain kinases, which are enzymes that play a pivotal role in cell signaling pathways . This inhibition can lead to changes in cellular processes and has potential therapeutic applications in treating diseases where kinase activity is dysregulated.
Cellular Effects
The effects of this compound on cells are profound. It influences cell function by modulating cell signaling pathways, gene expression, and cellular metabolism. Studies have shown that this compound can induce apoptosis (programmed cell death) in certain cancer cell lines by disrupting key signaling pathways . Additionally, it affects gene expression by altering the transcriptional activity of specific genes involved in cell proliferation and survival.
Molecular Mechanism
At the molecular level, this compound exerts its effects through binding interactions with target biomolecules. It can inhibit enzyme activity by binding to the active site of the enzyme, preventing substrate binding and subsequent catalytic activity . This inhibition can lead to downstream effects on cellular processes, including changes in gene expression and cellular metabolism. Furthermore, this compound can modulate the activity of transcription factors, thereby influencing the expression of genes involved in critical cellular functions.
Temporal Effects in Laboratory Settings
In laboratory settings, the effects of this compound can vary over time. The compound’s stability and degradation are important factors that influence its long-term effects on cellular function. Studies have shown that this compound remains stable under specific storage conditions but can degrade over time when exposed to certain environmental factors . Long-term exposure to this compound in in vitro and in vivo studies has demonstrated sustained effects on cellular processes, including prolonged inhibition of enzyme activity and alterations in gene expression.
Dosage Effects in Animal Models
The effects of this compound in animal models are dose-dependent. At lower doses, the compound can effectively inhibit target enzymes and modulate cellular processes without causing significant toxicity . At higher doses, this compound can exhibit toxic effects, including hepatotoxicity and nephrotoxicity. These adverse effects highlight the importance of determining the optimal dosage for therapeutic applications to minimize toxicity while maximizing efficacy.
Metabolic Pathways
This compound is involved in various metabolic pathways, interacting with enzymes and cofactors that facilitate its biotransformation . The compound can undergo phase I and phase II metabolic reactions, leading to the formation of metabolites that may have different biological activities. These metabolic pathways can influence the compound’s pharmacokinetics and pharmacodynamics, affecting its overall efficacy and safety profile.
Transport and Distribution
The transport and distribution of this compound within cells and tissues are mediated by specific transporters and binding proteins . The compound can be actively transported across cell membranes and distributed to various cellular compartments. Its localization and accumulation within specific tissues can influence its biological activity and therapeutic potential. Understanding the transport mechanisms of this compound is essential for optimizing its delivery and efficacy in therapeutic applications.
Subcellular Localization
This compound exhibits specific subcellular localization patterns that can affect its activity and function . The compound can be targeted to specific cellular compartments, such as the nucleus or mitochondria, through post-translational modifications or targeting signals. This subcellular localization can influence the compound’s interactions with biomolecules and its overall biological activity. Understanding the factors that govern the subcellular localization of this compound is crucial for elucidating its mechanism of action and optimizing its therapeutic potential.
Properties
IUPAC Name |
2,4-dichloro-5-fluoroquinazoline | |
---|---|---|
Source | PubChem | |
URL | https://pubchem.ncbi.nlm.nih.gov | |
Description | Data deposited in or computed by PubChem | |
InChI |
InChI=1S/C8H3Cl2FN2/c9-7-6-4(11)2-1-3-5(6)12-8(10)13-7/h1-3H | |
Source | PubChem | |
URL | https://pubchem.ncbi.nlm.nih.gov | |
Description | Data deposited in or computed by PubChem | |
InChI Key |
AXMYGHKVWFFYCR-UHFFFAOYSA-N | |
Source | PubChem | |
URL | https://pubchem.ncbi.nlm.nih.gov | |
Description | Data deposited in or computed by PubChem | |
Canonical SMILES |
C1=CC2=C(C(=C1)F)C(=NC(=N2)Cl)Cl | |
Source | PubChem | |
URL | https://pubchem.ncbi.nlm.nih.gov | |
Description | Data deposited in or computed by PubChem | |
Molecular Formula |
C8H3Cl2FN2 | |
Source | PubChem | |
URL | https://pubchem.ncbi.nlm.nih.gov | |
Description | Data deposited in or computed by PubChem | |
DSSTOX Substance ID |
DTXSID40652961 | |
Record name | 2,4-Dichloro-5-fluoroquinazoline | |
Source | EPA DSSTox | |
URL | https://comptox.epa.gov/dashboard/DTXSID40652961 | |
Description | DSSTox provides a high quality public chemistry resource for supporting improved predictive toxicology. | |
Molecular Weight |
217.02 g/mol | |
Source | PubChem | |
URL | https://pubchem.ncbi.nlm.nih.gov | |
Description | Data deposited in or computed by PubChem | |
CAS No. |
87611-00-5 | |
Record name | 2,4-Dichloro-5-fluoroquinazoline | |
Source | EPA DSSTox | |
URL | https://comptox.epa.gov/dashboard/DTXSID40652961 | |
Description | DSSTox provides a high quality public chemistry resource for supporting improved predictive toxicology. | |
Record name | 2,4-dichloro-5-fluoroquinazoline | |
Source | European Chemicals Agency (ECHA) | |
URL | https://echa.europa.eu/information-on-chemicals | |
Description | The European Chemicals Agency (ECHA) is an agency of the European Union which is the driving force among regulatory authorities in implementing the EU's groundbreaking chemicals legislation for the benefit of human health and the environment as well as for innovation and competitiveness. | |
Explanation | Use of the information, documents and data from the ECHA website is subject to the terms and conditions of this Legal Notice, and subject to other binding limitations provided for under applicable law, the information, documents and data made available on the ECHA website may be reproduced, distributed and/or used, totally or in part, for non-commercial purposes provided that ECHA is acknowledged as the source: "Source: European Chemicals Agency, http://echa.europa.eu/". Such acknowledgement must be included in each copy of the material. ECHA permits and encourages organisations and individuals to create links to the ECHA website under the following cumulative conditions: Links can only be made to webpages that provide a link to the Legal Notice page. | |
Retrosynthesis Analysis
AI-Powered Synthesis Planning: Our tool employs the Template_relevance Pistachio, Template_relevance Bkms_metabolic, Template_relevance Pistachio_ringbreaker, Template_relevance Reaxys, Template_relevance Reaxys_biocatalysis model, leveraging a vast database of chemical reactions to predict feasible synthetic routes.
One-Step Synthesis Focus: Specifically designed for one-step synthesis, it provides concise and direct routes for your target compounds, streamlining the synthesis process.
Accurate Predictions: Utilizing the extensive PISTACHIO, BKMS_METABOLIC, PISTACHIO_RINGBREAKER, REAXYS, REAXYS_BIOCATALYSIS database, our tool offers high-accuracy predictions, reflecting the latest in chemical research and data.
Strategy Settings
Precursor scoring | Relevance Heuristic |
---|---|
Min. plausibility | 0.01 |
Model | Template_relevance |
Template Set | Pistachio/Bkms_metabolic/Pistachio_ringbreaker/Reaxys/Reaxys_biocatalysis |
Top-N result to add to graph | 6 |
Feasible Synthetic Routes
Disclaimer and Information on In-Vitro Research Products
Please be aware that all articles and product information presented on BenchChem are intended solely for informational purposes. The products available for purchase on BenchChem are specifically designed for in-vitro studies, which are conducted outside of living organisms. In-vitro studies, derived from the Latin term "in glass," involve experiments performed in controlled laboratory settings using cells or tissues. It is important to note that these products are not categorized as medicines or drugs, and they have not received approval from the FDA for the prevention, treatment, or cure of any medical condition, ailment, or disease. We must emphasize that any form of bodily introduction of these products into humans or animals is strictly prohibited by law. It is essential to adhere to these guidelines to ensure compliance with legal and ethical standards in research and experimentation.