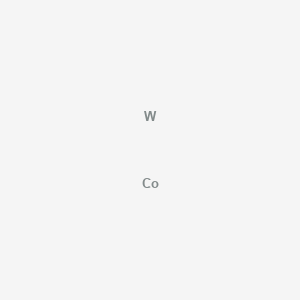
cobalt;tungsten
- Click on QUICK INQUIRY to receive a quote from our team of experts.
- With the quality product at a COMPETITIVE price, you can focus more on your research.
Overview
Description
Cobalt tungsten, often referred to as tungsten carbide cobalt, is a composite material known for its exceptional hardness, wear resistance, and durability. This compound is a blend of tungsten carbide (WC), a compound recognized for its extreme hardness, and cobalt (Co), which acts as a binder to hold the tungsten carbide particles together . The combination of these elements results in a material that is exceptionally strong and versatile, making it invaluable in various industrial applications.
Preparation Methods
Synthetic Routes and Reaction Conditions
The preparation of cobalt tungsten involves several steps:
Powder Preparation: Tungsten carbide powder is mixed with cobalt powder in precise ratios.
Compaction: The mixed powders are pressed into a desired shape using high pressure.
Sintering: The compacted material is heated to a temperature just below the melting point of cobalt.
Industrial Production Methods
Industrial production of cobalt tungsten often involves molten salt electrolysis, where tungsten carbide scrap is used as the sacrificial anode and NaCl–KCl salt as the electrolyte . This method allows for the efficient extraction of cobalt and tungsten from cemented carbide scraps, making it a sustainable approach to producing this compound .
Chemical Reactions Analysis
Types of Reactions
Cobalt tungsten undergoes various chemical reactions, including:
Oxidation: Cobalt tungsten can oxidize to form cobalt tungstate (CoWO4) and carbon dioxide (CO2) when exposed to oxygen.
Reduction: The compound can be reduced to its elemental forms under specific conditions.
Substitution: Cobalt tungsten can undergo substitution reactions where cobalt is replaced by other metals such as nickel or iron.
Common Reagents and Conditions
Oxidation: Requires oxygen (O2) and elevated temperatures.
Reduction: Involves the use of reducing agents such as hydrogen (H2) or carbon monoxide (CO).
Substitution: Typically occurs in the presence of other metal salts and under controlled temperature and pressure conditions.
Major Products Formed
Oxidation: Cobalt tungstate (CoWO4) and carbon dioxide (CO2).
Reduction: Elemental cobalt and tungsten.
Substitution: Various metal carbides depending on the substituting metal.
Scientific Research Applications
Cobalt tungsten has a wide range of scientific research applications:
Chemistry: Used as a catalyst in various chemical reactions, including water splitting for hydrogen production.
Biology: Cobalt compounds are used in biological systems for their antimicrobial properties.
Medicine: Cobalt tungsten is used in medical devices and implants due to its biocompatibility and durability.
Industry: Widely used in the manufacturing of cutting tools, mining equipment, and wear-resistant components.
Mechanism of Action
The mechanism by which cobalt tungsten exerts its effects involves the interaction of cobalt and tungsten ions with their molecular targets. Cobalt ions can interact with cellular components, leading to various biological effects, including antimicrobial activity . Tungsten ions can enhance the catalytic properties of the compound, making it effective in industrial applications .
Comparison with Similar Compounds
Similar Compounds
Nickel tungsten: Similar in structure but uses nickel as the binder instead of cobalt.
Iron tungsten: Uses iron as the binder and has different mechanical properties.
Titanium carbide: Another hard material used in cutting tools but with different chemical properties.
Uniqueness
Cobalt tungsten is unique due to its combination of extreme hardness, wear resistance, and toughness provided by the cobalt binder . This makes it more versatile and durable compared to other similar compounds .
Properties
CAS No. |
62431-64-5 |
---|---|
Molecular Formula |
CoW |
Molecular Weight |
242.77 g/mol |
IUPAC Name |
cobalt;tungsten |
InChI |
InChI=1S/Co.W |
InChI Key |
JPNWDVUTVSTKMV-UHFFFAOYSA-N |
Canonical SMILES |
[Co].[W] |
Origin of Product |
United States |
Disclaimer and Information on In-Vitro Research Products
Please be aware that all articles and product information presented on BenchChem are intended solely for informational purposes. The products available for purchase on BenchChem are specifically designed for in-vitro studies, which are conducted outside of living organisms. In-vitro studies, derived from the Latin term "in glass," involve experiments performed in controlled laboratory settings using cells or tissues. It is important to note that these products are not categorized as medicines or drugs, and they have not received approval from the FDA for the prevention, treatment, or cure of any medical condition, ailment, or disease. We must emphasize that any form of bodily introduction of these products into humans or animals is strictly prohibited by law. It is essential to adhere to these guidelines to ensure compliance with legal and ethical standards in research and experimentation.