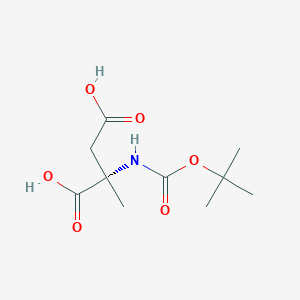
Boc-alpha-methyl-L-aspartic acid
Overview
Description
Boc-alpha-methyl-L-aspartic acid is a chemical compound with the molecular formula C10H17NO6 and a molecular weight of 247.24 g/mol. It is used as an intermediate in the preparation of modified alpha-amino acids .
Synthesis Analysis
The synthesis of Boc-alpha-methyl-L-aspartic acid involves the use of N-Boc-protected alpha-amino acid derivatives . A moisture-tolerant route to unprotected alpha/beta-amino acid N-carboxyanhydrides has been reported, which employs epoxy compounds as ultra-fast scavengers of hydrogen chloride to allow assisted ring-closure and prevent NCA from acid-catalyzed decomposition under moist conditions .
Molecular Structure Analysis
The molecular structure of Boc-alpha-methyl-L-aspartic acid consists of a Boc (tert-butoxycarbonyl) group attached to the nitrogen atom of the alpha-methyl-L-aspartic acid molecule. The Boc group serves as a protecting group for the amino group during peptide synthesis .
Chemical Reactions Analysis
Boc-alpha-methyl-L-aspartic acid can undergo various chemical reactions. For instance, it can be used to prepare isoxazoline glycoprotein IIb/IIIa antagonists and to synthesize glutamic acid analogs as potent inhibitors of leukotriene A4 hydrolase . The Boc group can be removed under acidic conditions, producing tert-butyl cations .
Physical And Chemical Properties Analysis
Boc-alpha-methyl-L-aspartic acid is a solid compound . Its physical and chemical properties are largely influenced by its molecular structure, particularly the presence of the Boc group and the alpha-methyl-L-aspartic acid moiety.
Scientific Research Applications
Synthesis and Chemical Applications
Boc-alpha-methyl-L-aspartic acid is used in the synthesis of peptides, especially in solid-phase peptide synthesis. Al-Obeidi et al. (2009) report the synthesis of β- and γ-fluorenylmethyl esters of Nα-Boc-L-aspartic acid, essential for side-chain to side-chain cyclizations during peptide synthesis (Al-Obeidi, Sanderson, & Hruby, 2009). Additionally, Wagner and Tilley (1990) synthesized a β-hydroxyaspartic acid derivative protected for incorporation into peptides by solid-phase synthesis, starting from N-Boc-(R)-serine (Wagner & Tilley, 1990).
Applications in Biological Studies
Research by Ndungu et al. (2004) demonstrates the use of N α -Boc protected aspartic acid in creating chiral β-allyl substituted aspartic acid, useful for constructing aspartic acid–glycine bicyclic dipeptide mimetics (Ndungu et al., 2004). Thaisrivongs et al. (1986) utilized Boc-Pro-Phe-N alpha-MeHis-Leu psi-[CHOHCH2]Val-Ile-Amp in a study of renin inhibitors, indicating its potential in drug discovery and development (Thaisrivongs et al., 1986).
Material Science and Nanotechnology
The utility of Boc-aspartic acid derivatives extends to material science as well. For instance, Subbalakshmi and Nagaraj (2014) explored the use of butyloxycarbonyl (Boc)-protected methyl esters of peptides for creating nanoparticles with potential applications in biology, such as in cell imaging and antimicrobial agents (Subbalakshmi & Nagaraj, 2014).
Mechanism of Action
Future Directions
Boc-alpha-methyl-L-aspartic acid, like other Boc-protected amino acids, has potential applications in the field of proteomics research . Its use in the synthesis of modified alpha-amino acids and various other compounds suggests that it could play a role in the development of new pharmaceuticals and therapeutic agents .
properties
IUPAC Name |
(2S)-2-methyl-2-[(2-methylpropan-2-yl)oxycarbonylamino]butanedioic acid | |
---|---|---|
Source | PubChem | |
URL | https://pubchem.ncbi.nlm.nih.gov | |
Description | Data deposited in or computed by PubChem | |
InChI |
InChI=1S/C10H17NO6/c1-9(2,3)17-8(16)11-10(4,7(14)15)5-6(12)13/h5H2,1-4H3,(H,11,16)(H,12,13)(H,14,15)/t10-/m0/s1 | |
Source | PubChem | |
URL | https://pubchem.ncbi.nlm.nih.gov | |
Description | Data deposited in or computed by PubChem | |
InChI Key |
WMDCRWFYQLYYCO-JTQLQIEISA-N | |
Source | PubChem | |
URL | https://pubchem.ncbi.nlm.nih.gov | |
Description | Data deposited in or computed by PubChem | |
Canonical SMILES |
CC(C)(C)OC(=O)NC(C)(CC(=O)O)C(=O)O | |
Source | PubChem | |
URL | https://pubchem.ncbi.nlm.nih.gov | |
Description | Data deposited in or computed by PubChem | |
Isomeric SMILES |
C[C@](CC(=O)O)(C(=O)O)NC(=O)OC(C)(C)C | |
Source | PubChem | |
URL | https://pubchem.ncbi.nlm.nih.gov | |
Description | Data deposited in or computed by PubChem | |
Molecular Formula |
C10H17NO6 | |
Source | PubChem | |
URL | https://pubchem.ncbi.nlm.nih.gov | |
Description | Data deposited in or computed by PubChem | |
Molecular Weight |
247.24 g/mol | |
Source | PubChem | |
URL | https://pubchem.ncbi.nlm.nih.gov | |
Description | Data deposited in or computed by PubChem | |
Retrosynthesis Analysis
AI-Powered Synthesis Planning: Our tool employs the Template_relevance Pistachio, Template_relevance Bkms_metabolic, Template_relevance Pistachio_ringbreaker, Template_relevance Reaxys, Template_relevance Reaxys_biocatalysis model, leveraging a vast database of chemical reactions to predict feasible synthetic routes.
One-Step Synthesis Focus: Specifically designed for one-step synthesis, it provides concise and direct routes for your target compounds, streamlining the synthesis process.
Accurate Predictions: Utilizing the extensive PISTACHIO, BKMS_METABOLIC, PISTACHIO_RINGBREAKER, REAXYS, REAXYS_BIOCATALYSIS database, our tool offers high-accuracy predictions, reflecting the latest in chemical research and data.
Strategy Settings
Precursor scoring | Relevance Heuristic |
---|---|
Min. plausibility | 0.01 |
Model | Template_relevance |
Template Set | Pistachio/Bkms_metabolic/Pistachio_ringbreaker/Reaxys/Reaxys_biocatalysis |
Top-N result to add to graph | 6 |
Feasible Synthetic Routes
Disclaimer and Information on In-Vitro Research Products
Please be aware that all articles and product information presented on BenchChem are intended solely for informational purposes. The products available for purchase on BenchChem are specifically designed for in-vitro studies, which are conducted outside of living organisms. In-vitro studies, derived from the Latin term "in glass," involve experiments performed in controlled laboratory settings using cells or tissues. It is important to note that these products are not categorized as medicines or drugs, and they have not received approval from the FDA for the prevention, treatment, or cure of any medical condition, ailment, or disease. We must emphasize that any form of bodily introduction of these products into humans or animals is strictly prohibited by law. It is essential to adhere to these guidelines to ensure compliance with legal and ethical standards in research and experimentation.