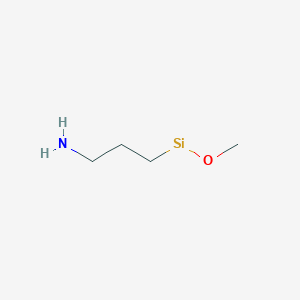
3-Aminopropylmethoxysilane
- Click on QUICK INQUIRY to receive a quote from our team of experts.
- With the quality product at a COMPETITIVE price, you can focus more on your research.
Overview
Description
3-Aminopropyltrimethoxysilane (APTS, CAS 13822-56-5) is an organosilane with the molecular formula C₆H₁₇NO₃Si. It features a primary amine group (-NH₂) and three methoxy (-OCH₃) groups bonded to a silicon atom. APTS is widely used as a coupling agent to enhance adhesion between organic polymers (e.g., epoxides, polyamides) and inorganic substrates (e.g., glass, metals) . Its methoxy groups hydrolyze in the presence of moisture to form silanol (-SiOH), which bonds to inorganic surfaces, while the amine group reacts with organic matrices, improving mechanical and chemical resistance in composites, coatings, and adhesives .
Preparation Methods
Synthetic Routes and Reaction Conditions: 3-Aminopropylmethoxysilane can be synthesized through the reaction of 3-aminopropylamine with methoxysilanes. The typical reaction involves the following steps:
Reaction Setup: The reaction is carried out in a solvent such as toluene or xylene.
Addition of Reagents: 3-aminopropylamine is added to the methoxysilane under an inert atmosphere.
Reaction Conditions: The mixture is heated to a temperature range of 80-120°C and stirred for several hours.
Purification: The product is purified through distillation or recrystallization to obtain pure this compound.
Industrial Production Methods: In industrial settings, the production of this compound involves large-scale reactors and optimized reaction conditions to ensure high yield and purity. The process may include continuous flow reactors and advanced purification techniques to meet industrial standards.
Chemical Reactions Analysis
Types of Reactions: 3-Aminopropylmethoxysilane undergoes various chemical reactions, including:
Hydrolysis: The methoxy groups can hydrolyze in the presence of water to form silanols.
Condensation: The silanols can further condense to form siloxane bonds, leading to the formation of cross-linked networks.
Amination: The amino group can react with various electrophiles, such as aldehydes and ketones, to form imines or amines.
Common Reagents and Conditions:
Hydrolysis: Water or aqueous solutions under acidic or basic conditions.
Condensation: Catalysts such as acids or bases to promote siloxane bond formation.
Amination: Electrophiles like aldehydes, ketones, or isocyanates under mild to moderate temperatures.
Major Products Formed:
Hydrolysis and Condensation: Siloxane networks or films.
Amination: Imines or secondary amines, depending on the electrophile used.
Scientific Research Applications
3-Aminopropyltrimethoxysilane (APTMS) is a versatile organosilane with a wide range of applications across various industries, including adhesives, sealants, surface treatments, and health applications . It acts as an adhesion/cohesion promoter, sealant, and intermediate in manufacturing processes .
Scientific Research Applications
Biocomposites
APTMS is used as a silane coupling agent to improve the adhesion between different components in biocomposites. For example, it enhances the compatibility between chemically modified oil palm mesocarp fiber (OPMF) and polybutylene succinate (PBS) in biocomposites . The incorporation of 2 wt.% APTMS into a SNOPMF/PBS biocomposite improved tensile, flexural, and impact strengths by 16%, 30%, and 15%, respectively . It also enhanced resistance to water uptake and thickness swelling by 34% and 49%, respectively .
Surface Modification
APTMS is employed to modify the surface of various materials, enhancing their properties and functionality . It has been used to derivatize the surface of mesoporous alumina for iodine removal in radioactive waste management . APTMS can be directly polymerized on glass surfaces using a plasma jet, modifying the surface properties .
Health Applications
In the health sector, APTMS is used as a surface modifier of fibers to improve nitric oxide release properties for hypoxia/reoxygenation injury treatment . It is also utilized as a modifier in dental fillers to minimize shrinkage stress, thereby improving the lifetime and performance of dental materials .
Industrial and Environmental Applications
APTMS is used as a modifier component of biogenic silica membranes to enhance the separation properties of CO2/N2 .
Nanoparticle Coating
APTMS is utilized to coat nanoparticles (NPs), which are then used in applications such as chemical sensors and cosmetic products .
Data Table: Applications of 3-Aminopropyltrimethoxysilane
Case Studies
Biocomposite Improvement: A study on oil palm mesocarp fiber (OPMF) and polybutylene succinate (PBS) biocomposites demonstrated that incorporating 2 wt.% APTMS improved tensile, flexural, and impact strengths, as well as water resistance and thickness swelling .
Surface Modification for Environmental Applications: APTMS has been used to modify mesoporous alumina for the removal of iodine under radioactive settings, contributing to the safe geological disposal of radioactive nuclei .
Health Application in Dental Fillers: APTMS is employed as a modifier in dental fillers to minimize shrinkage stress experienced by unmodified silica materials, thus improving the lifetime and performance of the filler materials .
Mechanism of Action
The primary mechanism of action of 3-aminopropylmethoxysilane involves the hydrolysis of methoxy groups to form silanols, which can then condense to form siloxane bonds. The amino group provides reactivity towards various electrophiles, enabling the functionalization of surfaces and the formation of covalent bonds with other molecules. This dual functionality makes it a valuable compound for surface modification and crosslinking applications .
Comparison with Similar Compounds
Structural and Functional Differences
The table below compares APTS with three structurally related silanes:
Key Insights:
- Alkoxy Group Impact: Methoxy groups (APTS) hydrolyze faster than ethoxy (APTES), enabling rapid silanol formation for quick adhesion . Ethoxy groups in APTES provide slower curing, reducing stress in thermal cycling applications (e.g., aerospace coatings) .
- Amine Substitution : MAPTS’s methylated amine reduces hydrogen bonding, minimizing self-condensation and improving dispersion in hydrophobic polymers like polyethylene .
- Chain Length and Functionality : AEAPTMS’s diamine structure enhances crosslinking density, improving chemical resistance in extreme environments (e.g., marine coatings) .
Hydrogen Bonding and Hydrolysis Behavior
Computational spectroscopy studies reveal that APTS forms stronger intermolecular hydrogen bonds (N-H···O) compared to APTES and MAPTS. The methoxy groups in APTS generate shorter hydrogen bonds (2.08 Å) than ethoxy in APTES (2.15 Å), leading to higher surface energy and reactivity . MAPTS’s methyl group disrupts hydrogen bonding, reducing its interfacial adhesion but improving compatibility with non-polar substrates .
Hydrolysis Rates:
- APTS : Hydrolyzes within minutes under ambient humidity, ideal for fast-setting adhesives .
- APTES : Requires hours for full hydrolysis, suitable for controlled curing in high-temperature applications .
Thermal and Mechanical Performance
- APTS : Exhibits moderate thermal stability (decomposes at ~250°C), limiting use in high-temperature environments. However, its rapid bonding is advantageous in room-temperature composites .
- APTES : Superior thermal resistance (stable up to ~300°C) due to ethoxy groups’ stability, making it preferred in automotive and electronic coatings .
- AEAPTMS : High crosslink density from diamine groups improves mechanical strength (tensile strength >50 MPa) in epoxy composites .
Q & A
Basic Research Questions
Q. What are the standard protocols for synthesizing 3-Aminopropylmethoxysilane in laboratory settings?
this compound is typically synthesized via hydrolysis-condensation reactions under controlled conditions. A common method involves reacting aminopropyl precursors with methoxysilane groups in anhydrous solvents (e.g., toluene) to minimize premature hydrolysis. The reaction requires inert atmospheres (e.g., nitrogen or argon) to prevent moisture interference. Post-synthesis purification via vacuum distillation or column chromatography is critical to remove unreacted monomers and byproducts .
Q. Which characterization techniques are essential for confirming the structure and purity of this compound?
Key techniques include:
- Nuclear Magnetic Resonance (NMR) : For verifying molecular structure (e.g., ¹H NMR peaks at δ 0.6–1.2 ppm for Si-CH₂ groups and δ 2.6–3.0 ppm for NH₂ protons) .
- Fourier-Transform Infrared Spectroscopy (FTIR) : To confirm Si-O-Si (~1050 cm⁻¹) and NH₂ (~3300 cm⁻¹) bonding .
- Elemental Analysis : For quantifying carbon, hydrogen, and nitrogen content to validate purity (>97%) .
Q. What are the primary research applications of this compound in materials science?
This silane is widely used as:
- Surface Coupling Agent : Enhances adhesion between organic polymers and inorganic substrates (e.g., glass, silica nanoparticles) in nanocomposites .
- Biomaterial Functionalization : Modifies surfaces for protein immobilization or cell culture substrates due to its amine reactivity .
- Electronic Materials : Serves as a dielectric layer precursor in semiconductor fabrication .
Q. What safety protocols are mandatory for handling this compound in laboratory settings?
- Personal Protective Equipment (PPE) : Tightly fitting safety goggles, nitrile gloves, and chemical-resistant lab coats .
- Ventilation : Use fume hoods to avoid inhalation of vapors, which can cause respiratory irritation .
- Spill Management : Absorb spills with inert materials (e.g., sand) and dispose of as hazardous waste .
Advanced Research Questions
Q. How can researchers optimize reaction conditions for this compound-mediated surface modification to enhance substrate adhesion?
Key parameters include:
- pH Control : Maintain mildly acidic conditions (pH 4–5) to promote silanol (Si-OH) formation on substrates .
- Concentration : Use 1–5% (v/v) silane solutions to avoid multilayer formation and ensure monolayer grafting .
- Curing Temperature : Post-grafting annealing at 80–120°C improves crosslinking and stability .
Q. How should contradictory data regarding the thermal stability of this compound-derived layers be analyzed?
Discrepancies may arise from:
- Environmental Humidity : Higher moisture levels accelerate hydrolytic degradation of siloxane bonds. Use thermogravimetric analysis (TGA) under controlled humidity to assess stability .
- Substrate Composition : Inorganic substrates (e.g., SiO₂) enhance thermal resistance compared to organic matrices. Compare data using X-ray photoelectron spectroscopy (XPS) to track bond degradation .
Q. What advanced analytical methods are recommended for quantifying residual amine groups after silane grafting?
- XPS : Measures nitrogen atomic % on surfaces to estimate amine density .
- Fluorescamine Assay : Reacts with primary amines to produce fluorescent adducts, enabling quantitative analysis via fluorescence spectroscopy .
- Acid-Base Titration : For bulk samples, titrate with HCl to determine free amine content .
Q. How can researchers mitigate aggregation issues in this compound-functionalized nanoparticles?
- Sonication : Use probe sonication during functionalization to disrupt particle clusters .
- Solvent Selection : Polar aprotic solvents (e.g., DMF) improve dispersion stability compared to non-polar solvents .
- Surface Charge Modulation : Adjust pH to maintain zeta potential > ±30 mV for electrostatic stabilization .
Q. Methodological Considerations
Q. What experimental controls are critical when studying this compound’s reactivity in aqueous environments?
- Blank Substrates : Include unmodified substrates to distinguish silane-specific effects .
- Hydrolysis Time Series : Monitor reaction progress at 0, 1, 3, and 24 hours to identify equilibrium conditions .
- Stability Controls : Store samples under nitrogen to compare hydrolytic degradation rates .
Q. How should researchers validate the reproducibility of silane grafting efficiency across batches?
Properties
Molecular Formula |
C4H11NOSi |
---|---|
Molecular Weight |
117.22 g/mol |
InChI |
InChI=1S/C4H11NOSi/c1-6-7-4-2-3-5/h2-5H2,1H3 |
InChI Key |
GRAKIAFZHSPONK-UHFFFAOYSA-N |
Canonical SMILES |
CO[Si]CCCN |
Origin of Product |
United States |
Disclaimer and Information on In-Vitro Research Products
Please be aware that all articles and product information presented on BenchChem are intended solely for informational purposes. The products available for purchase on BenchChem are specifically designed for in-vitro studies, which are conducted outside of living organisms. In-vitro studies, derived from the Latin term "in glass," involve experiments performed in controlled laboratory settings using cells or tissues. It is important to note that these products are not categorized as medicines or drugs, and they have not received approval from the FDA for the prevention, treatment, or cure of any medical condition, ailment, or disease. We must emphasize that any form of bodily introduction of these products into humans or animals is strictly prohibited by law. It is essential to adhere to these guidelines to ensure compliance with legal and ethical standards in research and experimentation.