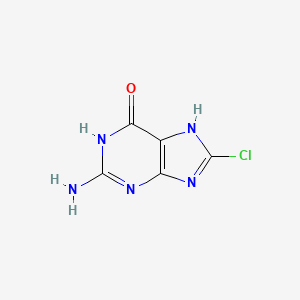
8-Chloroguanine
Overview
Description
8-Chloroguanine is a halogenated derivative of guanine, a purine nucleobase found in DNA and RNA. This compound is formed when guanine undergoes chlorination, typically under conditions of oxidative stress or exposure to reactive halogen species such as hypochlorous acid. This compound is of significant interest due to its potential role in mutagenesis and carcinogenesis, particularly in the context of chronic inflammation .
Mechanism of Action
Target of Action
- 8-Chloroguanine (ClG) primarily targets genomic DNA. ClG is a major lesion formed by the attack of hypochlorous acid (released by myeloperoxidase during inflammation) on guanine residues in DNA . It is implicated in mutagenesis and carcinogenesis.
Mode of Action
Action Environment
Biochemical Analysis
Biochemical Properties
8-Chloroguanine plays a crucial role in biochemical reactions, particularly in the context of DNA damage and repair mechanisms. It interacts with various enzymes and proteins involved in DNA replication and repair. For instance, human DNA polymerase β (polβ) has been shown to incorporate this compound into DNA, albeit with reduced efficiency compared to normal guanine . This interaction can lead to mutagenic replication, as this compound can form base pairs with cytosine and guanine using its anti and syn conformers, respectively . Additionally, this compound can be recognized and processed by DNA repair enzymes, which attempt to excise and replace the damaged base.
Cellular Effects
This compound has profound effects on cellular processes. It can induce mutations during DNA replication, leading to genomic instability. This compound is particularly relevant in the context of chronic inflammation, where reactive halogen species such as hypochlorous acid are produced by immune cells . These reactive species can chlorinate guanine residues in DNA, forming this compound and promoting G to C transversion mutations . Such mutations can disrupt normal cell signaling pathways, alter gene expression, and affect cellular metabolism, potentially leading to carcinogenesis.
Molecular Mechanism
At the molecular level, this compound exerts its effects through several mechanisms. It can form Hoogsteen base pairs with incoming nucleotides during DNA synthesis, which facilitates mutagenic replication . The presence of this compound in DNA can also hinder the normal function of DNA polymerases, leading to replication errors and strand breaks. Furthermore, this compound can be recognized by DNA repair enzymes, which may attempt to excise the damaged base, leading to further genomic instability if the repair process is error-prone .
Temporal Effects in Laboratory Settings
In laboratory settings, the effects of this compound can change over time. The compound is relatively stable at room temperature but is recommended to be stored in a freezer for long-term stability . Over time, this compound can degrade, which may influence its biological activity and the outcomes of experimental studies. Long-term exposure to this compound in cell cultures can lead to cumulative DNA damage, affecting cellular function and viability .
Dosage Effects in Animal Models
The effects of this compound vary with different dosages in animal models. At low doses, it may cause subtle DNA damage that can be repaired by cellular mechanisms. At higher doses, this compound can induce significant genomic instability, leading to toxic or adverse effects . Studies have shown that high doses of this compound can lead to increased mutation rates and potentially carcinogenic outcomes .
Metabolic Pathways
This compound is involved in metabolic pathways related to DNA damage and repair. It interacts with enzymes such as DNA polymerases and DNA glycosylases, which are involved in the recognition and excision of damaged bases . The presence of this compound can affect metabolic flux by altering the normal progression of DNA replication and repair processes .
Transport and Distribution
Within cells, this compound is transported and distributed primarily through its incorporation into DNA. It can be recognized by DNA repair proteins, which may transport it to specific cellular compartments for processing . The distribution of this compound within tissues can vary depending on the extent of exposure to reactive halogen species and the efficiency of DNA repair mechanisms .
Subcellular Localization
This compound is primarily localized within the nucleus, where it is incorporated into genomic DNA. Its activity and function are influenced by its subcellular localization, as it can interfere with DNA replication and repair processes within the nucleus . The presence of this compound in specific DNA regions can lead to localized genomic instability and affect the expression of nearby genes .
Preparation Methods
Synthetic Routes and Reaction Conditions: The synthesis of 8-Chloroguanine typically involves the chlorination of guanine. One common method includes the reaction of guanine with hypochlorous acid or other chlorinating agents under controlled conditions. The reaction is usually carried out in an aqueous medium at a specific pH to ensure selective chlorination at the 8-position of the guanine molecule .
Industrial Production Methods: Industrial production of this compound may involve similar chlorination processes but on a larger scale. The use of continuous flow reactors and optimized reaction conditions can enhance yield and purity. Additionally, purification steps such as recrystallization or chromatography are employed to isolate the desired product .
Chemical Reactions Analysis
Types of Reactions: 8-Chloroguanine undergoes various chemical reactions, including:
Oxidation: It can be further oxidized to form other derivatives.
Reduction: Reduction reactions can convert this compound back to guanine or other reduced forms.
Substitution: The chlorine atom at the 8-position can be substituted with other nucleophiles, leading to the formation of different derivatives.
Common Reagents and Conditions:
Oxidation: Common oxidizing agents include hydrogen peroxide and potassium permanganate.
Reduction: Reducing agents such as sodium borohydride or catalytic hydrogenation can be used.
Substitution: Nucleophiles like amines, thiols, or hydroxyl groups can react with this compound under appropriate conditions.
Major Products: The major products formed from these reactions depend on the specific reagents and conditions used. For example, substitution reactions can yield various 8-substituted guanine derivatives .
Scientific Research Applications
8-Chloroguanine has several scientific research applications:
Chemistry: It is used as a model compound to study halogenation reactions and the effects of halogenation on nucleobase properties.
Biology: Researchers investigate its role in DNA damage and repair mechanisms, particularly in the context of oxidative stress and inflammation.
Medicine: Studies focus on its potential mutagenic and carcinogenic effects, contributing to our understanding of cancer development.
Industry: It is used in the development of diagnostic tools and assays to detect oxidative DNA damage.
Comparison with Similar Compounds
8-Oxoguanine: Another oxidized derivative of guanine, known for its role in oxidative DNA damage.
5-Chlorouracil: A halogenated derivative of uracil, used in similar studies of DNA damage and repair.
2-Chloroadenine: A chlorinated adenine derivative, also studied for its mutagenic properties.
Uniqueness of 8-Chloroguanine: this compound is unique due to its specific formation under conditions of oxidative stress and its distinct mutagenic potential. Unlike 8-Oxoguanine, which primarily causes G to T transversions, this compound is associated with G to C transversions, highlighting its unique impact on DNA replication fidelity .
Properties
IUPAC Name |
2-amino-8-chloro-1,7-dihydropurin-6-one | |
---|---|---|
Source | PubChem | |
URL | https://pubchem.ncbi.nlm.nih.gov | |
Description | Data deposited in or computed by PubChem | |
InChI |
InChI=1S/C5H4ClN5O/c6-4-8-1-2(9-4)10-5(7)11-3(1)12/h(H4,7,8,9,10,11,12) | |
Source | PubChem | |
URL | https://pubchem.ncbi.nlm.nih.gov | |
Description | Data deposited in or computed by PubChem | |
InChI Key |
YCFWZXAEOXKNHL-UHFFFAOYSA-N | |
Source | PubChem | |
URL | https://pubchem.ncbi.nlm.nih.gov | |
Description | Data deposited in or computed by PubChem | |
Canonical SMILES |
C12=C(N=C(NC1=O)N)N=C(N2)Cl | |
Source | PubChem | |
URL | https://pubchem.ncbi.nlm.nih.gov | |
Description | Data deposited in or computed by PubChem | |
Molecular Formula |
C5H4ClN5O | |
Source | PubChem | |
URL | https://pubchem.ncbi.nlm.nih.gov | |
Description | Data deposited in or computed by PubChem | |
DSSTOX Substance ID |
DTXSID40176531 | |
Record name | Guanine, 8-chloro- | |
Source | EPA DSSTox | |
URL | https://comptox.epa.gov/dashboard/DTXSID40176531 | |
Description | DSSTox provides a high quality public chemistry resource for supporting improved predictive toxicology. | |
Molecular Weight |
185.57 g/mol | |
Source | PubChem | |
URL | https://pubchem.ncbi.nlm.nih.gov | |
Description | Data deposited in or computed by PubChem | |
CAS No. |
22052-03-5 | |
Record name | Guanine, 8-chloro- | |
Source | ChemIDplus | |
URL | https://pubchem.ncbi.nlm.nih.gov/substance/?source=chemidplus&sourceid=0022052035 | |
Description | ChemIDplus is a free, web search system that provides access to the structure and nomenclature authority files used for the identification of chemical substances cited in National Library of Medicine (NLM) databases, including the TOXNET system. | |
Record name | Guanine, 8-chloro- | |
Source | EPA DSSTox | |
URL | https://comptox.epa.gov/dashboard/DTXSID40176531 | |
Description | DSSTox provides a high quality public chemistry resource for supporting improved predictive toxicology. | |
Retrosynthesis Analysis
AI-Powered Synthesis Planning: Our tool employs the Template_relevance Pistachio, Template_relevance Bkms_metabolic, Template_relevance Pistachio_ringbreaker, Template_relevance Reaxys, Template_relevance Reaxys_biocatalysis model, leveraging a vast database of chemical reactions to predict feasible synthetic routes.
One-Step Synthesis Focus: Specifically designed for one-step synthesis, it provides concise and direct routes for your target compounds, streamlining the synthesis process.
Accurate Predictions: Utilizing the extensive PISTACHIO, BKMS_METABOLIC, PISTACHIO_RINGBREAKER, REAXYS, REAXYS_BIOCATALYSIS database, our tool offers high-accuracy predictions, reflecting the latest in chemical research and data.
Strategy Settings
Precursor scoring | Relevance Heuristic |
---|---|
Min. plausibility | 0.01 |
Model | Template_relevance |
Template Set | Pistachio/Bkms_metabolic/Pistachio_ringbreaker/Reaxys/Reaxys_biocatalysis |
Top-N result to add to graph | 6 |
Feasible Synthetic Routes
Q1: How does 8-chloroguanine impact DNA replication and what are the potential consequences?
A: this compound (ClG) is a major DNA lesion primarily caused by inflammation-induced hypochlorous acid attack on guanine bases. [] This modification can disrupt normal DNA replication. Studies using human DNA polymerase β showed that while ClG preferentially pairs with cytosine, it can also form mutagenic base pairs with guanine during DNA replication. [] This mispairing primarily occurs due to ClG's ability to adopt a syn conformation, facilitating Hoogsteen base pairing with an incoming guanine. [] This misincorporation can lead to G to C transversion mutations, highlighting the promutagenic nature of ClG. []
Q2: What makes this compound a significant subject of study in the context of inflammation and disease?
A: this compound is a marker of hypochlorite (HOCl) activity in biological systems. [] HOCl, a potent oxidant, is produced by the enzyme myeloperoxidase, primarily released by activated neutrophils and macrophages during inflammation. [, ] Elevated levels of myeloperoxidase and this compound have been linked to various diseases, including atherosclerosis, kidney diseases, and chronic inflammation. [] Therefore, understanding the formation, repair mechanisms, and mutagenic potential of this compound is crucial for deciphering its role in inflammation-driven disease development.
Q3: Are there any potential therapeutic strategies being explored that target this compound?
A: While the provided research papers don't directly focus on therapeutic strategies for this compound, one paper discusses novel nucleotide analogues as potential antiviral agents. [] Although not directly targeting this compound, these analogues are structurally similar to natural nucleosides and act as precursor molecules. [] Upon phosphorylation within cells, they can potentially interfere with viral DNA replication. [] Further research is needed to explore if similar strategies can be applied to target this compound formation or its downstream effects.
Disclaimer and Information on In-Vitro Research Products
Please be aware that all articles and product information presented on BenchChem are intended solely for informational purposes. The products available for purchase on BenchChem are specifically designed for in-vitro studies, which are conducted outside of living organisms. In-vitro studies, derived from the Latin term "in glass," involve experiments performed in controlled laboratory settings using cells or tissues. It is important to note that these products are not categorized as medicines or drugs, and they have not received approval from the FDA for the prevention, treatment, or cure of any medical condition, ailment, or disease. We must emphasize that any form of bodily introduction of these products into humans or animals is strictly prohibited by law. It is essential to adhere to these guidelines to ensure compliance with legal and ethical standards in research and experimentation.