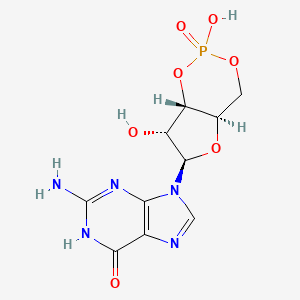
Cyclic guanosine monophosphate
Overview
Description
Cyclic guanosine monophosphate (cGMP) is a cyclic nucleotide second messenger derived from guanosine triphosphate (GTP) via enzymatic catalysis by guanylate cyclase (GC). Its structure features a 3',5'-cyclic phosphate linkage, distinguishing it from linear nucleotides like GTP or GDP. cGMP plays pivotal roles in diverse physiological processes, including vasodilation, phototransduction, and cellular differentiation .
Preparation Methods
Enzymatic Synthesis of cGMP
The enzymatic synthesis of cGMP primarily involves the catalytic action of guanylate cyclase enzymes, which convert guanosine triphosphate (GTP) into cGMP. This method is highly specific and mimics physiological processes.
- Soluble guanylate cyclase (sGC) is the enzyme responsible for converting GTP to cGMP in vivo, activated by nitric oxide (NO) signaling.
- The enzymatic reaction is conducted under mild conditions, typically at physiological pH and temperature, ensuring high stereospecificity.
- Enzymatic assays for cGMP production use substrates and cofactors, with reaction monitoring by bioluminescent or luminescence-based detection systems to quantify cGMP formation.
- Reaction termination often involves inhibitors such as IBMX to halt phosphodiesterase activity, preserving cGMP for measurement.
- High specificity and stereochemical fidelity.
- Mild reaction conditions.
- Direct relevance to physiological pathways.
- Enzyme availability and stability.
- Scale-up challenges for industrial synthesis.
Representative Experimental Setup:
Component | Concentration/Condition | Purpose |
---|---|---|
Guanylate cyclase enzyme | 0.1 mg/mL BSA stabilized | Catalyzes GTP to cGMP conversion |
cGMP substrate | 100 nM cGMP in PDE reaction buffer | Reaction initiation |
IBMX | 1.25 mM in PDE reaction buffer | Phosphodiesterase inhibitor |
Incubation | 25 °C for 25 minutes | Reaction progression |
Detection | Luminescence via Nano-Glo Luciferase assay | Quantification of cGMP |
Source: Adapted from ACS Publications on bioluminescent assay for cGMP monitoring.
Chemical Synthesis of cGMP and Analogues
Chemical synthesis of cGMP involves multi-step organic synthesis, often starting from protected guanosine derivatives. The goal is to form the 3′,5′-cyclic phosphate linkage with high regio- and stereoselectivity.
The H-Phosphonate Approach for Cyclic Guanosine Monophosphorothioate (cGMPS)
Process Summary:
- The H-phosphonate monoester of the nucleoside is cyclized to form a 3′,5′-cyclic H-phosphonate diester.
- Subsequent sulfurization converts the H-phosphonate diester into the phosphorothioate analogue.
- A silyl protection strategy is employed to selectively protect the 2′ and 5′ hydroxyl groups, minimizing side reactions and improving selectivity.
- The process achieves a diastereomeric ratio of approximately 9:1 favoring the R P-diastereomer, which is the desired active form.
- Purification is achieved predominantly by crystallization, avoiding chromatographic steps, which facilitates scale-up.
Step | Reagents/Conditions | Outcome/Notes |
---|---|---|
Silyl Protection | Triisopropylsilyl chloride, selective for 2′,5′-OH | >80% selectivity for 2′,5′-protection |
Cyclization | Coupling agent (e.g., pivaloyl chloride) | Formation of cyclic H-phosphonate intermediate |
Sulfurization | Sulfur and triethylamine | Conversion to cyclic phosphorothioate |
Purification | Crystallization | >99.9% purity, 13.8% total yield on scale-up |
- The process was scaled to produce 125 grams of the phosphorothioate analogue with high purity.
- Total isolated yield was approximately 13.8%.
Parameter | Value/Condition |
---|---|
Starting material | 310 g (0.37 mol) of protected nucleoside |
Coupling agent | Pivaloyl chloride (1.5 equiv) |
Sulfurization agents | Sulfur (1.5 equiv), triethylamine (1.5 equiv) |
Diastereomeric ratio (R:S) | 9:1 |
Purity (HPLC) | >99.9% |
Total yield | 13.8% |
Source: Adapted from ACS Organic Process Research & Development on cGMPS synthesis.
Alternative Chemical Methods
- Chlorination–amidation–Stec sequence starting from cyclic adenosine monophosphate has been reported but requires chromatographic purification and yields only one diastereomer.
- Protection strategies are critical to avoid side products such as 2′,3′-cyclic phosphates and to control regioselectivity.
Comparative Analysis of Preparation Methods
Preparation Method | Advantages | Limitations | Applications |
---|---|---|---|
Enzymatic synthesis | High specificity, physiological relevance | Enzyme stability, scale-up challenges | Research assays, physiological studies |
Chemical synthesis (H-phosphonate route) | High stereoselectivity, scalable, chromatography-free | Moderate overall yield, multi-step process | Pharmaceutical production, analog synthesis |
Chemical synthesis (Chlorination–amidation) | Stereoselective for one diastereomer | Requires chromatography, limited scalability | Specialized analog production |
Research Findings and Notes
- The H-phosphonate approach provides a viable route for producing cyclic guanosine monophosphorothioate analogues with high purity and selectivity, critical for drug development targeting retinal neurodegenerations.
- Enzymatic methods remain the gold standard for physiological relevance but are less practical for large-scale synthesis.
- Protection of hydroxyl groups on the ribose ring is essential to direct cyclization and avoid side reactions.
- Crystallization-based purification enhances scalability and reduces costs compared to chromatographic methods.
- The stereochemistry of the phosphorus center is crucial for biological activity, necessitating careful control during synthesis.
Chemical Reactions Analysis
Cyclic guanosine monophosphate undergoes various chemical reactions, including:
Oxidation: this compound can be oxidized to produce guanosine monophosphate.
Reduction: It can be reduced to form guanosine.
Substitution: this compound can undergo substitution reactions where the phosphate group is replaced by other functional groups. Common reagents used in these reactions include oxidizing agents like hydrogen peroxide and reducing agents like sodium borohydride.
Scientific Research Applications
Cardiovascular Health
Cyclic guanosine monophosphate plays a significant role in cardiovascular physiology. It mediates vasodilation and counteracts maladaptive cardiac remodeling.
- Heart Failure : Studies indicate that elevated plasma levels of cGMP are associated with lower risks of heart failure with preserved ejection fraction (HFpEF) and atherosclerotic cardiovascular disease (ASCVD) . The cGMP signaling pathway is being targeted for new therapeutic strategies in heart failure management.
- Mechanisms of Action : cGMP activates protein kinases that promote smooth muscle relaxation and inhibit platelet aggregation . This pathway is crucial for maintaining vascular homeostasis and preventing thrombotic events.
Application | Mechanism | Clinical Relevance |
---|---|---|
Heart Failure | Enhances left ventricular relaxation | Potential biomarker for heart failure risk |
Vasodilation | Activates smooth muscle relaxation | Therapeutic target for hypertension |
Neurobiology
This compound is implicated in neuroplasticity and mood regulation.
- Major Depressive Disorder : Research suggests that cGMP signaling influences neurogenesis and synaptic plasticity, particularly in brain regions associated with mood regulation . Enhancing cGMP levels may provide therapeutic benefits in treating major depressive disorder.
- Mechanistic Insights : The activation of guanylate cyclase by neurotransmitters increases cGMP levels, which subsequently modulate gene expression and neuronal function .
Application | Mechanism | Clinical Relevance |
---|---|---|
Neuroplasticity | Amplifies neurotransmitter signals | Potential treatment for depression |
Synaptic Function | Modulates gene expression | Enhances cognitive functions |
Infectious Disease Management
This compound also plays a role in the pathogenesis of certain infectious diseases.
- Pathogen Evasion : Certain pathogens like Enterotoxigenic Escherichia coli utilize cGMP to evade host immune responses. The toxin produced by ETEC increases intracellular cGMP levels, which can suppress effective immune responses .
- Therapeutic Implications : Understanding the role of cGMP in pathogen interactions could lead to novel therapeutic strategies aimed at enhancing host defenses against infections.
Application | Mechanism | Clinical Relevance |
---|---|---|
Immune Evasion | Induces cGMP production in host cells | Target for vaccine development |
Pathogen Interaction | Modulates host signaling pathways | Potential for new antimicrobial therapies |
Case Study 1: Heart Failure Risk Assessment
A study involving participants from the Atherosclerosis Risk in Communities (ARIC) demonstrated that higher plasma cGMP levels correlate with a reduced risk of developing HFpEF and other cardiovascular conditions . This finding supports the potential use of plasma cGMP as a biomarker for assessing heart failure risk.
Case Study 2: Neuroplasticity Enhancement
Research on chronic antidepressant treatments has shown that these medications can elevate cGMP levels in the hippocampus, leading to improved neuroplasticity and cognitive function . This highlights the therapeutic potential of targeting the cGMP pathway in treating mood disorders.
Mechanism of Action
Cyclic guanosine monophosphate exerts its effects by activating intracellular protein kinases in response to the binding of membrane-impermeable peptide hormones to the external cell surface. This activation leads to the relaxation of smooth muscle tissues, vasodilation, and increased blood flow. In the photoreceptors of the mammalian eye, this compound controls the opening and closing of sodium ion channels, which is crucial for visual signal transduction .
Comparison with Similar Compounds
Key Features of cGMP:
- Synthesis : Two guanylate cyclase isoforms regulate cGMP production: soluble GC (sGC, activated by nitric oxide) and particulate GC (pGC, activated by natriuretic peptides or bacterial enterotoxins) .
- Degradation : Phosphodiesterases (PDEs), particularly PDE5 and PDE6, hydrolyze cGMP to 5'-GMP, terminating its signaling .
- Functions: Mediates nitric oxide (NO)-induced vasodilation by relaxing vascular smooth muscle . Regulates phototransduction in retinal rod cells . Modulates neural stem cell differentiation and synaptic plasticity .
Comparison with Cyclic Adenosine Monophosphate (cAMP)
Structural and Functional Differences
Feature | cGMP | cAMP |
---|---|---|
Precursor | GTP | ATP |
Synthesis Enzyme | Guanylate cyclase (GC) | Adenylate cyclase (AC) |
Primary Receptors | Protein kinase G (PKG) | Protein kinase A (PKA) |
Key Roles | Vasodilation, vision, apoptosis | Glycogenolysis, cardiac contractility, hormone secretion |
Regulatory Roles in Disease
- Cancer : In Morris hepatomas, cGMP levels are significantly lower than in healthy liver tissue, whereas cAMP remains unchanged. This suggests tumor-specific dysregulation of cGMP signaling .
- Hematopoiesis: cAMP inhibits proliferation of normal granulocyte-macrophage progenitors (CFU-Cs), while cGMP enhances colony formation, highlighting opposing roles in myeloid cell regulation .
- Kidney Fibrosis : Both nucleotides modulate renal fibrosis, but cAMP promotes fibroblast activation, while cGMP counteracts fibrotic pathways via PKG .
Independent Regulation
Radioimmunoassay studies confirm cAMP and cGMP are independently regulated. Hormonal stimuli (e.g., glucagon) elevate cAMP without affecting cGMP, and adrenalectomy increases muscle cGMP without altering cAMP .
Comparison with Other Cyclic Nucleotides
Cyclic Dinucleotides: c-di-GMP and cGAMP
Feature | cGMP | c-di-GMP | cGAMP |
---|---|---|---|
Structure | Mononucleotide (3',5'-cyclic) | Dinucleotide (3',5'-linked) | Heterodinucleotide (2',3'- or 3',3'-linked) |
Synthesis | Guanylate cyclase | Diguanylate cyclase | cGAS enzyme (from ATP/GTP) |
Function | Vasodilation, neural signaling | Bacterial biofilm formation | Immune response (STING pathway activation) |
Therapeutic Target | PDE5 inhibitors (sildenafil) | Antibiofilm agents | Anticancer/antiviral therapies |
- c-di-GMP : Bacterial second messenger regulating biofilm formation and virulence. Unlike cGMP, it lacks direct roles in mammalian systems .
- cGAMP : Activates the STING pathway to induce interferon production, contrasting with cGMP's vascular and neural roles .
Clinical and Therapeutic Implications
cGMP in Disease Pathogenesis
- Cardiovascular Disease : Reduced cGMP levels correlate with heart failure and fibrosis. Sacubitril/valsartan enhances cGMP by inhibiting NPR-A degradation .
- Diabetes: Acute hyperglycemia elevates serum cGMP, but chronic hyperglycemia impairs NO-cGMP signaling, contributing to vascular dysfunction .
PDE Inhibitors
- PDE5 Inhibitors : Sildenafil increases cGMP by blocking degradation, improving erectile dysfunction and pulmonary hypertension .
- Dual PDE3/4 Inhibitors : Target both cAMP and cGMP in inflammatory diseases, demonstrating synergistic anti-inflammatory effects .
Data Tables
Table 1: cGMP and cAMP Levels in Morris Hepatomas vs. Liver
Tissue | cGMP (pmol/mg protein) | cAMP (pmol/mg protein) |
---|---|---|
Normal Liver | 0.45 ± 0.12 | 1.20 ± 0.30 |
Morris Hepatoma | 0.18 ± 0.05* | 1.15 ± 0.25 |
*Significantly lower than liver (p < 0.01).
Table 2: Serum cGMP Concentrations in Clinical Conditions
Group | cGMP (pmol/mL, Median ± IQR) |
---|---|
Healthy (CON) | 152 ± 86 |
Liver Fibrosis | 91 ± 22* |
Fibrosis + Sil | 168 ± 153 |
*Significantly reduced in fibrosis (p < 0.05).
Biological Activity
Cyclic guanosine monophosphate (cGMP) is a crucial intracellular second messenger involved in various physiological processes across different biological systems. Its synthesis and degradation are tightly regulated, and it plays significant roles in cardiovascular health, immune responses, and plant biology. This article explores the biological activity of cGMP, supported by recent research findings, case studies, and data tables.
Overview of cGMP Functionality
cGMP is synthesized from guanosine triphosphate (GTP) by guanylate cyclase enzymes, which can be activated by nitric oxide (NO) or natriuretic peptides. The signaling pathways mediated by cGMP are essential for various cellular functions:
- Vasodilation : cGMP induces relaxation of smooth muscle cells, contributing to vasodilation and regulating blood pressure.
- Cardiac Function : It plays a role in myocardial relaxation and counteracts maladaptive cardiac remodeling.
- Neurotransmission : In the nervous system, cGMP is involved in synaptic plasticity and memory formation.
- Plant Responses : In plants, cGMP is implicated in responses to stress and developmental processes.
Cardiovascular Activity of cGMP
Recent studies highlight the cardioprotective effects of cGMP in cardiovascular diseases (CVD). It has been shown that increased levels of cGMP can lead to beneficial outcomes in heart failure and pulmonary arterial hypertension.
Table 1: Effects of cGMP on Cardiovascular Health
Function | Mechanism | Outcome |
---|---|---|
Vasodilation | Activation of protein kinase G (PKG) | Reduced blood pressure |
Myocardial relaxation | Modulation of calcium handling | Improved cardiac output |
Counteracting hypertrophy | Inhibition of growth factor signaling | Prevention of heart failure progression |
Case Study : A study involving patients with heart failure demonstrated that enhancing cGMP signaling through pharmacological agents resulted in improved cardiac function and reduced hospitalization rates for heart-related issues .
Immune System Modulation
cGMP also plays a pivotal role in the immune response. The STING (Stimulator of Interferon Genes) pathway, which is activated by cyclic GMP-AMP (cGAMP), triggers immune responses upon detection of cytoplasmic DNA.
Table 2: Role of cGMP in Immune Responses
Immune Function | Mechanism | Outcome |
---|---|---|
Cytokine production | Activation of NF-κB and IRF3 | Enhanced inflammatory response |
Apoptosis induction | Modulation of cell death pathways | Regulation of T cell responses |
Research Finding : In systemic autoinflammatory disease (SAVI), constitutive activation of STING leads to persistent immune activation, highlighting the importance of cGMP in regulating immune homeostasis .
Plant Biology and Stress Responses
In plants, cGMP is synthesized in response to nitric oxide during abiotic and biotic stress. It regulates stomatal closure, root development, and defense mechanisms against pathogens.
Table 3: Role of cGMP in Plant Stress Responses
Stress Type | cGMP Role | Outcome |
---|---|---|
Pathogen attack | Activation of defense gene expression | Enhanced resistance |
Osmotic stress | Stomatal closure | Reduced water loss |
Root development | Regulation of adventitious root formation | Improved nutrient uptake |
Case Study : Research on Arabidopsis thaliana revealed that mutants lacking guanylate cyclase showed impaired stomatal closure under NO treatment, indicating the critical role of the NO-cGMP pathway in plant stress responses .
Q & A
Basic Research Questions
Q. How can researchers overcome challenges in detecting low tissue concentrations of cyclic guanosine monophosphate (cGMP) in physiological studies?
this compound (cGMP) concentrations in tissues are often low due to rapid turnover and compartmentalization. To address this, use radioimmunoassays (RIA) with high-affinity antibodies or liquid chromatography-mass spectrometry (LC-MS) for enhanced sensitivity and specificity . Avoid older colorimetric methods (e.g., Folin phenol reagent), which lack the sensitivity required for cGMP quantification .
Q. What experimental approaches validate the role of cGMP in smooth muscle relaxation?
Utilize ex vivo tissue bath assays with vascular smooth muscle strips. Activate soluble guanylate cyclase (sGC) using nitric oxide (NO) donors (e.g., sodium nitroprusside) to elevate cGMP levels, then measure relaxation via force transducers. Confirm cGMP involvement by pre-treating tissues with sGC inhibitors (e.g., ODQ) or cGMP-dependent protein kinase (PKG) blockers (e.g., KT5823) .
Q. How do researchers distinguish between cytosolic and membrane-associated guanylate cyclase isoforms in functional studies?
Employ subcellular fractionation (e.g., differential centrifugation) to isolate cytosolic (sGC) and particulate (pGC) isoforms. Validate isoform-specific activation: sGC responds to NO donors, while pGC is activated by atriopeptins or heat-stable enterotoxins. Use knockout models or siRNA silencing to dissect isoform contributions .
Advanced Research Questions
Q. What methodologies resolve contradictions in cGMP signaling pathways when experimental outcomes conflict with established models?
Apply phosphoproteomics to identify PKG substrate phosphorylation patterns under varying conditions. Combine knockout/transgenic models (e.g., PKG-deficient mice) with real-time cGMP biosensors (e.g., FRET-based cGMP indicators) to monitor spatiotemporal signaling dynamics. Cross-validate findings using kinase activity assays (e.g., radioactive phosphate incorporation) .
Q. How can researchers assess cGMP cross-talk with cyclic adenosine monophosphate (cAMP) in shared regulatory pathways?
Use competitive binding assays (e.g., equilibrium dialysis) to quantify interactions between cGMP/cAMP and shared targets like the cAMP receptor protein (CRP). Analyze cooperativity parameters (e.g., α values) under varying ionic strengths to map competitive vs. synergistic effects. Pair with FRET-based dual-reporter systems to monitor both nucleotides simultaneously .
Q. What strategies evaluate the therapeutic potential of cGMP pathway modulation in vascular diseases?
Conduct translational studies using biomarker panels (e.g., urinary cGMP excretion [UcGMPV] and natriuretic peptides) to assess endothelial dysfunction. Test cGMP-elevating agents (e.g., sGC stimulators like riociguat) in preclinical models of hypertension or atherosclerosis, correlating outcomes with PKG activity assays and histopathological analysis .
Q. How can researchers dissect the role of cGMP in immune activation via the cGAS-STING pathway?
Use cGAS/STING knockout cells to isolate cGMP-specific effects in innate immunity. Measure cyclic dinucleotide levels (e.g., 2'3'-cGAMP) via LC-MS and monitor interferon-β production. Employ STING agonists/antagonists to validate cGMP’s role in modulating immune-active microenvironments, particularly in cancer immunotherapy contexts .
Q. Methodological Considerations
- cGMP Quantification : Prioritize LC-MS for absolute quantification in complex matrices (e.g., tumor microenvironments) .
- Isoform-Specific Probes : Use NO-insensitive pGC mutants to isolate pGC activity in heterologous systems .
- Data Interpretation : Account for tissue-specific cGMP compartmentalization using compartmentalized biosensors (e.g., targeted PKG FRET probes) .
Properties
IUPAC Name |
9-[(4aR,6R,7R,7aS)-2,7-dihydroxy-2-oxo-4a,6,7,7a-tetrahydro-4H-furo[3,2-d][1,3,2]dioxaphosphinin-6-yl]-2-amino-1H-purin-6-one | |
---|---|---|
Source | PubChem | |
URL | https://pubchem.ncbi.nlm.nih.gov | |
Description | Data deposited in or computed by PubChem | |
InChI |
InChI=1S/C10H12N5O7P/c11-10-13-7-4(8(17)14-10)12-2-15(7)9-5(16)6-3(21-9)1-20-23(18,19)22-6/h2-3,5-6,9,16H,1H2,(H,18,19)(H3,11,13,14,17)/t3-,5-,6-,9-/m1/s1 | |
Source | PubChem | |
URL | https://pubchem.ncbi.nlm.nih.gov | |
Description | Data deposited in or computed by PubChem | |
InChI Key |
ZOOGRGPOEVQQDX-UUOKFMHZSA-N | |
Source | PubChem | |
URL | https://pubchem.ncbi.nlm.nih.gov | |
Description | Data deposited in or computed by PubChem | |
Canonical SMILES |
C1C2C(C(C(O2)N3C=NC4=C3N=C(NC4=O)N)O)OP(=O)(O1)O | |
Source | PubChem | |
URL | https://pubchem.ncbi.nlm.nih.gov | |
Description | Data deposited in or computed by PubChem | |
Isomeric SMILES |
C1[C@@H]2[C@H]([C@H]([C@@H](O2)N3C=NC4=C3N=C(NC4=O)N)O)OP(=O)(O1)O | |
Source | PubChem | |
URL | https://pubchem.ncbi.nlm.nih.gov | |
Description | Data deposited in or computed by PubChem | |
Molecular Formula |
C10H12N5O7P | |
Source | PubChem | |
URL | https://pubchem.ncbi.nlm.nih.gov | |
Description | Data deposited in or computed by PubChem | |
DSSTOX Substance ID |
DTXSID8040646 | |
Record name | Cyclic guanosine monophosphate | |
Source | EPA DSSTox | |
URL | https://comptox.epa.gov/dashboard/DTXSID8040646 | |
Description | DSSTox provides a high quality public chemistry resource for supporting improved predictive toxicology. | |
Molecular Weight |
345.21 g/mol | |
Source | PubChem | |
URL | https://pubchem.ncbi.nlm.nih.gov | |
Description | Data deposited in or computed by PubChem | |
Physical Description |
Solid | |
Record name | Cyclic GMP | |
Source | Human Metabolome Database (HMDB) | |
URL | http://www.hmdb.ca/metabolites/HMDB0001314 | |
Description | The Human Metabolome Database (HMDB) is a freely available electronic database containing detailed information about small molecule metabolites found in the human body. | |
Explanation | HMDB is offered to the public as a freely available resource. Use and re-distribution of the data, in whole or in part, for commercial purposes requires explicit permission of the authors and explicit acknowledgment of the source material (HMDB) and the original publication (see the HMDB citing page). We ask that users who download significant portions of the database cite the HMDB paper in any resulting publications. | |
CAS No. |
7665-99-8 | |
Record name | cGMP | |
Source | CAS Common Chemistry | |
URL | https://commonchemistry.cas.org/detail?cas_rn=7665-99-8 | |
Description | CAS Common Chemistry is an open community resource for accessing chemical information. Nearly 500,000 chemical substances from CAS REGISTRY cover areas of community interest, including common and frequently regulated chemicals, and those relevant to high school and undergraduate chemistry classes. This chemical information, curated by our expert scientists, is provided in alignment with our mission as a division of the American Chemical Society. | |
Explanation | The data from CAS Common Chemistry is provided under a CC-BY-NC 4.0 license, unless otherwise stated. | |
Record name | Cyclic GMP | |
Source | ChemIDplus | |
URL | https://pubchem.ncbi.nlm.nih.gov/substance/?source=chemidplus&sourceid=0007665998 | |
Description | ChemIDplus is a free, web search system that provides access to the structure and nomenclature authority files used for the identification of chemical substances cited in National Library of Medicine (NLM) databases, including the TOXNET system. | |
Record name | Cyclic GMP | |
Source | DrugBank | |
URL | https://www.drugbank.ca/drugs/DB02315 | |
Description | The DrugBank database is a unique bioinformatics and cheminformatics resource that combines detailed drug (i.e. chemical, pharmacological and pharmaceutical) data with comprehensive drug target (i.e. sequence, structure, and pathway) information. | |
Explanation | Creative Common's Attribution-NonCommercial 4.0 International License (http://creativecommons.org/licenses/by-nc/4.0/legalcode) | |
Record name | Cyclic guanosine monophosphate | |
Source | EPA DSSTox | |
URL | https://comptox.epa.gov/dashboard/DTXSID8040646 | |
Description | DSSTox provides a high quality public chemistry resource for supporting improved predictive toxicology. | |
Record name | CYCLIC GMP | |
Source | FDA Global Substance Registration System (GSRS) | |
URL | https://gsrs.ncats.nih.gov/ginas/app/beta/substances/H2D2X058MU | |
Description | The FDA Global Substance Registration System (GSRS) enables the efficient and accurate exchange of information on what substances are in regulated products. Instead of relying on names, which vary across regulatory domains, countries, and regions, the GSRS knowledge base makes it possible for substances to be defined by standardized, scientific descriptions. | |
Explanation | Unless otherwise noted, the contents of the FDA website (www.fda.gov), both text and graphics, are not copyrighted. They are in the public domain and may be republished, reprinted and otherwise used freely by anyone without the need to obtain permission from FDA. Credit to the U.S. Food and Drug Administration as the source is appreciated but not required. | |
Record name | Cyclic GMP | |
Source | Human Metabolome Database (HMDB) | |
URL | http://www.hmdb.ca/metabolites/HMDB0001314 | |
Description | The Human Metabolome Database (HMDB) is a freely available electronic database containing detailed information about small molecule metabolites found in the human body. | |
Explanation | HMDB is offered to the public as a freely available resource. Use and re-distribution of the data, in whole or in part, for commercial purposes requires explicit permission of the authors and explicit acknowledgment of the source material (HMDB) and the original publication (see the HMDB citing page). We ask that users who download significant portions of the database cite the HMDB paper in any resulting publications. | |
Retrosynthesis Analysis
AI-Powered Synthesis Planning: Our tool employs the Template_relevance Pistachio, Template_relevance Bkms_metabolic, Template_relevance Pistachio_ringbreaker, Template_relevance Reaxys, Template_relevance Reaxys_biocatalysis model, leveraging a vast database of chemical reactions to predict feasible synthetic routes.
One-Step Synthesis Focus: Specifically designed for one-step synthesis, it provides concise and direct routes for your target compounds, streamlining the synthesis process.
Accurate Predictions: Utilizing the extensive PISTACHIO, BKMS_METABOLIC, PISTACHIO_RINGBREAKER, REAXYS, REAXYS_BIOCATALYSIS database, our tool offers high-accuracy predictions, reflecting the latest in chemical research and data.
Strategy Settings
Precursor scoring | Relevance Heuristic |
---|---|
Min. plausibility | 0.01 |
Model | Template_relevance |
Template Set | Pistachio/Bkms_metabolic/Pistachio_ringbreaker/Reaxys/Reaxys_biocatalysis |
Top-N result to add to graph | 6 |
Feasible Synthetic Routes
Disclaimer and Information on In-Vitro Research Products
Please be aware that all articles and product information presented on BenchChem are intended solely for informational purposes. The products available for purchase on BenchChem are specifically designed for in-vitro studies, which are conducted outside of living organisms. In-vitro studies, derived from the Latin term "in glass," involve experiments performed in controlled laboratory settings using cells or tissues. It is important to note that these products are not categorized as medicines or drugs, and they have not received approval from the FDA for the prevention, treatment, or cure of any medical condition, ailment, or disease. We must emphasize that any form of bodily introduction of these products into humans or animals is strictly prohibited by law. It is essential to adhere to these guidelines to ensure compliance with legal and ethical standards in research and experimentation.