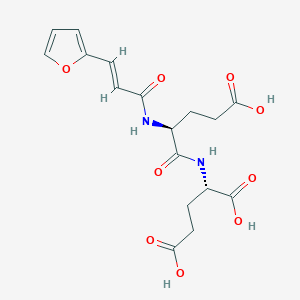
FA-Glu-Glu-OH
Overview
Description
FA-Glu-Glu-OH is a useful research compound. Its molecular formula is C17H20N2O9 and its molecular weight is 396.3 g/mol. The purity is usually 95%.
BenchChem offers high-quality this compound suitable for many research applications. Different packaging options are available to accommodate customers' requirements. Please inquire for more information about this compound including the price, delivery time, and more detailed information at info@benchchem.com.
Mechanism of Action
Target of Action
FA-Glu-Glu-OH is a dipeptide that can be used as an intermediate in peptide synthesis . It is the preferred substrate of human and zebrafish carboxypeptidase O . Carboxypeptidase O is a glycosylphosphatidylinositol-anchored intestinal peptidase with acidic amino acid specificity .
Biochemical Pathways
This compound, being a glutamine derivative, may potentially influence glutamine metabolism. Glutamine is an important amino acid that supplies carbon and nitrogen to fuel biosynthesis . It plays a versatile role in cell metabolism, participating in the tri-carboxylic acid (TCA) cycle supplementation and the biosynthesis of nucleotides, glutathione (GSH), and other nonessential amino acids . Thus, any compound affecting glutamine metabolism could have significant downstream effects on these biochemical pathways.
Pharmacokinetics
As a dipeptide, it is expected to be absorbed in the gastrointestinal tract, distributed throughout the body, metabolized into its constituent amino acids, and excreted via the kidneys .
Result of Action
The molecular and cellular effects of this compound’s action are likely to be diverse, given the wide-ranging roles of glutamine in cellular metabolism. For instance, glutamine supports mitochondrial oxidative metabolism when pyruvate derived from glucose is converted into lactate and secreted . Therefore, this compound could potentially influence these processes.
Biochemical Analysis
Biochemical Properties
FA-Glu-Glu-OH plays a significant role in biochemical reactions, particularly in the synthesis of peptides. It interacts with various enzymes, proteins, and other biomolecules. One notable interaction is with carboxypeptidase O, a glycosylphosphatidylinositol-anchored intestinal peptidase that has specificity for acidic amino acids . This interaction is crucial for the hydrolysis of peptide bonds involving glutamic acid residues. Additionally, this compound can be used as a substrate for proteases, which further process the dipeptide into its constituent amino acids .
Cellular Effects
This compound influences various cellular processes, including cell signaling pathways, gene expression, and cellular metabolism. In particular, the compound has been shown to affect the glutamine metabolic pathway, which is essential for cell biosynthesis and bioenergetics . By participating in this pathway, this compound can impact the production of nucleotides, amino acids, and other critical metabolites. This, in turn, influences cell function and viability, especially in rapidly proliferating cells such as cancer cells .
Molecular Mechanism
The molecular mechanism of this compound involves its interactions with specific enzymes and transporters. For instance, the compound can be hydrolyzed by proteases, releasing free glutamic acid residues that participate in various metabolic pathways . Additionally, this compound may interact with vesicular glutamate transporters, which regulate the packaging and release of glutamate in synaptic vesicles . These interactions are essential for maintaining glutamate homeostasis and preventing excitotoxicity in neuronal cells.
Temporal Effects in Laboratory Settings
In laboratory settings, the effects of this compound can change over time due to its stability and degradation. Studies have shown that the compound is relatively stable under standard storage conditions, but its activity may decrease over prolonged periods . Long-term exposure to this compound in cell cultures can lead to alterations in cellular function, including changes in gene expression and metabolic activity . These temporal effects are important to consider when designing experiments and interpreting results.
Dosage Effects in Animal Models
The effects of this compound can vary with different dosages in animal models. At low doses, the compound may enhance cellular metabolism and promote cell growth . At higher doses, this compound can exhibit toxic effects, leading to cell death and tissue damage . These dosage-dependent effects highlight the importance of optimizing the concentration of this compound in experimental studies to achieve the desired outcomes without causing adverse effects.
Metabolic Pathways
This compound is involved in several metabolic pathways, including the glutamine metabolic pathway. This pathway is crucial for the synthesis of nucleotides, amino acids, and other essential metabolites . This compound can be converted into glutamic acid, which then participates in the tricarboxylic acid (TCA) cycle and other metabolic processes . The compound’s involvement in these pathways can influence metabolic flux and the levels of various metabolites within cells.
Transport and Distribution
The transport and distribution of this compound within cells and tissues are mediated by specific transporters and binding proteins. Vesicular glutamate transporters, for example, play a key role in the uptake and release of the compound in neuronal cells . Additionally, this compound can be transported across cell membranes via amino acid transporters, facilitating its distribution to different cellular compartments . These transport mechanisms are essential for the compound’s localization and function within cells.
Subcellular Localization
This compound is localized in various subcellular compartments, including the cytoplasm and synaptic vesicles. The compound’s activity and function can be influenced by its subcellular localization, as it interacts with different biomolecules in specific compartments . For instance, in neuronal cells, this compound is concentrated in synaptic vesicles, where it plays a role in neurotransmitter release and synaptic signaling . Understanding the subcellular localization of this compound is crucial for elucidating its biological functions and potential therapeutic applications.
Properties
IUPAC Name |
(2S)-2-[[(2S)-4-carboxy-2-[[(E)-3-(furan-2-yl)prop-2-enoyl]amino]butanoyl]amino]pentanedioic acid | |
---|---|---|
Source | PubChem | |
URL | https://pubchem.ncbi.nlm.nih.gov | |
Description | Data deposited in or computed by PubChem | |
InChI |
InChI=1S/C17H20N2O9/c20-13(6-3-10-2-1-9-28-10)18-11(4-7-14(21)22)16(25)19-12(17(26)27)5-8-15(23)24/h1-3,6,9,11-12H,4-5,7-8H2,(H,18,20)(H,19,25)(H,21,22)(H,23,24)(H,26,27)/b6-3+/t11-,12-/m0/s1 | |
Source | PubChem | |
URL | https://pubchem.ncbi.nlm.nih.gov | |
Description | Data deposited in or computed by PubChem | |
InChI Key |
SWSOTQMOAWBLFA-NDYVEPOPSA-N | |
Source | PubChem | |
URL | https://pubchem.ncbi.nlm.nih.gov | |
Description | Data deposited in or computed by PubChem | |
Canonical SMILES |
C1=COC(=C1)C=CC(=O)NC(CCC(=O)O)C(=O)NC(CCC(=O)O)C(=O)O | |
Source | PubChem | |
URL | https://pubchem.ncbi.nlm.nih.gov | |
Description | Data deposited in or computed by PubChem | |
Isomeric SMILES |
C1=COC(=C1)/C=C/C(=O)N[C@@H](CCC(=O)O)C(=O)N[C@@H](CCC(=O)O)C(=O)O | |
Source | PubChem | |
URL | https://pubchem.ncbi.nlm.nih.gov | |
Description | Data deposited in or computed by PubChem | |
Molecular Formula |
C17H20N2O9 | |
Source | PubChem | |
URL | https://pubchem.ncbi.nlm.nih.gov | |
Description | Data deposited in or computed by PubChem | |
Molecular Weight |
396.3 g/mol | |
Source | PubChem | |
URL | https://pubchem.ncbi.nlm.nih.gov | |
Description | Data deposited in or computed by PubChem | |
Retrosynthesis Analysis
AI-Powered Synthesis Planning: Our tool employs the Template_relevance Pistachio, Template_relevance Bkms_metabolic, Template_relevance Pistachio_ringbreaker, Template_relevance Reaxys, Template_relevance Reaxys_biocatalysis model, leveraging a vast database of chemical reactions to predict feasible synthetic routes.
One-Step Synthesis Focus: Specifically designed for one-step synthesis, it provides concise and direct routes for your target compounds, streamlining the synthesis process.
Accurate Predictions: Utilizing the extensive PISTACHIO, BKMS_METABOLIC, PISTACHIO_RINGBREAKER, REAXYS, REAXYS_BIOCATALYSIS database, our tool offers high-accuracy predictions, reflecting the latest in chemical research and data.
Strategy Settings
Precursor scoring | Relevance Heuristic |
---|---|
Min. plausibility | 0.01 |
Model | Template_relevance |
Template Set | Pistachio/Bkms_metabolic/Pistachio_ringbreaker/Reaxys/Reaxys_biocatalysis |
Top-N result to add to graph | 6 |
Feasible Synthetic Routes
Disclaimer and Information on In-Vitro Research Products
Please be aware that all articles and product information presented on BenchChem are intended solely for informational purposes. The products available for purchase on BenchChem are specifically designed for in-vitro studies, which are conducted outside of living organisms. In-vitro studies, derived from the Latin term "in glass," involve experiments performed in controlled laboratory settings using cells or tissues. It is important to note that these products are not categorized as medicines or drugs, and they have not received approval from the FDA for the prevention, treatment, or cure of any medical condition, ailment, or disease. We must emphasize that any form of bodily introduction of these products into humans or animals is strictly prohibited by law. It is essential to adhere to these guidelines to ensure compliance with legal and ethical standards in research and experimentation.