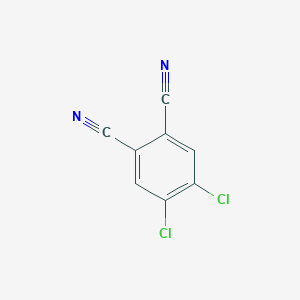
4,5-Dichlorophthalonitrile
Overview
Description
4,5-Dichlorophthalonitrile, also known as 4,5-dichloro-1,2-dicyanobenzene, is a phthalonitrile derivative . It has been synthesized from 4,5-dichloro-1,2-benzenedicarboxamide and characterized by 1H, 13C-NMR, and IR .
Synthesis Analysis
The synthesis of 4,5-Dichlorophthalonitrile involves several steps. Starting with 4,5-dichlorophthalic acid as the raw material, the compound is synthesized through dehydration by acetic anhydride, imidization by formamide, ammonolysis by ammonia solution, and dehydration by phosphorus oxychloride . This process can be simplified using a one-pot method, which also improves the reaction yield .Molecular Structure Analysis
The molecular formula of 4,5-Dichlorophthalonitrile is C8H2Cl2N2 . It has an average mass of 197.021 Da and a monoisotopic mass of 195.959503 Da .Chemical Reactions Analysis
4,5-Dichlorophthalonitrile undergoes base-catalyzed nucleophilic aromatic substitution reactions with O-, S- nucleophiles, and acidic -CH containing compounds to form corresponding phthalonitrile derivatives . These derivatives are precursors in the synthesis of phthalocyanines .Physical And Chemical Properties Analysis
4,5-Dichlorophthalonitrile has a density of 1.5±0.1 g/cm3, a boiling point of 312.4±42.0 °C at 760 mmHg, and a vapor pressure of 0.0±0.7 mmHg at 25°C . It has a molar refractivity of 45.5±0.4 cm3, a polar surface area of 48 Å2, and a molar volume of 132.4±5.0 cm3 .Scientific Research Applications
Synthesis of Phthalocyanines
4,5-Dichlorophthalonitrile is used in the synthesis of phthalocyanines . Phthalocyanines are a class of compounds that are widely used in various fields such as organic electronics, photovoltaics, and catalysis due to their excellent thermal and chemical stability, high electron affinity, and strong absorption in the near-infrared region.
Synthesis of 4,5-bis(3,4-dimethoxyphenyl)phthalonitrile
This compound is used as a reactant in the synthesis of 4,5-bis(3,4-dimethoxyphenyl)phthalonitrile . The resulting product has potential applications in the field of organic electronics due to its unique electronic properties.
Synthesis of 4,5-bis(2,6-dimethoxyphenoxy)phthalonitrile
4,5-Dichlorophthalonitrile is also used in the synthesis of 4,5-bis(2,6-dimethoxyphenoxy)phthalonitrile . This compound is of interest in the field of organic semiconductors.
Synthesis of 4,5-diphenoxyphthalonitrile
Another application of 4,5-Dichlorophthalonitrile is in the synthesis of 4,5-diphenoxyphthalonitrile . This compound is a key intermediate in the synthesis of various organic compounds.
Base Catalyzed Nucleophilic Aromatic Substitution Reactions
4,5-Dichlorophthalonitrile undergoes base catalyzed nucleophilic aromatic substitution reactions with O-, S- nucleophiles and acidic -CH containing compounds . This makes it a valuable compound in organic synthesis.
Precursor in the Synthesis of Other Compounds
Due to its unique structure, 4,5-Dichlorophthalonitrile serves as a precursor in the synthesis of a variety of other compounds . This makes it a versatile compound in the field of organic chemistry.
Safety and Hazards
Mechanism of Action
Target of Action
4,5-Dichlorophthalonitrile, also known as 4,5-dichloro-1,2-dicyanobenzene, is a phthalonitrile derivative It’s known that phthalonitriles are precursors in the synthesis of phthalocyanines , which are used in various applications such as solar cell materials, nonlinear optical materials, and optical disc recording materials .
Mode of Action
4,5-Dichlorophthalonitrile undergoes base-catalyzed nucleophilic aromatic substitution reactions with O-, S- nucleophiles and acidic -CH containing compounds to form corresponding phthalonitrile derivatives . These derivatives are then used in the synthesis of phthalocyanines .
Biochemical Pathways
It’s known that the compound plays a crucial role in the synthesis of phthalocyanines , which are involved in various biochemical pathways due to their wide range of applications .
Pharmacokinetics
It’s known that the compound has high gi absorption and is bbb permeant . It’s also known to be a CYP1A2 inhibitor . The compound has a log P (iLOGP) of 1.55, indicating its lipophilicity , which can impact its bioavailability.
Result of Action
As a precursor in the synthesis of phthalocyanines , the compound indirectly contributes to the various molecular and cellular effects brought about by phthalocyanines in their respective applications .
Action Environment
It’s recommended that the compound be stored in a sealed container in a dry room at room temperature , suggesting that moisture and temperature could potentially affect its stability.
properties
IUPAC Name |
4,5-dichlorobenzene-1,2-dicarbonitrile | |
---|---|---|
Source | PubChem | |
URL | https://pubchem.ncbi.nlm.nih.gov | |
Description | Data deposited in or computed by PubChem | |
InChI |
InChI=1S/C8H2Cl2N2/c9-7-1-5(3-11)6(4-12)2-8(7)10/h1-2H | |
Source | PubChem | |
URL | https://pubchem.ncbi.nlm.nih.gov | |
Description | Data deposited in or computed by PubChem | |
InChI Key |
SRIJSZQFAMLVQV-UHFFFAOYSA-N | |
Source | PubChem | |
URL | https://pubchem.ncbi.nlm.nih.gov | |
Description | Data deposited in or computed by PubChem | |
Canonical SMILES |
C1=C(C(=CC(=C1Cl)Cl)C#N)C#N | |
Source | PubChem | |
URL | https://pubchem.ncbi.nlm.nih.gov | |
Description | Data deposited in or computed by PubChem | |
Molecular Formula |
C8H2Cl2N2 | |
Source | PubChem | |
URL | https://pubchem.ncbi.nlm.nih.gov | |
Description | Data deposited in or computed by PubChem | |
DSSTOX Substance ID |
DTXSID00357485 | |
Record name | 4,5-Dichlorophthalonitrile | |
Source | EPA DSSTox | |
URL | https://comptox.epa.gov/dashboard/DTXSID00357485 | |
Description | DSSTox provides a high quality public chemistry resource for supporting improved predictive toxicology. | |
Molecular Weight |
197.02 g/mol | |
Source | PubChem | |
URL | https://pubchem.ncbi.nlm.nih.gov | |
Description | Data deposited in or computed by PubChem | |
Product Name |
4,5-Dichlorophthalonitrile | |
CAS RN |
139152-08-2 | |
Record name | 4,5-Dichlorophthalonitrile | |
Source | EPA DSSTox | |
URL | https://comptox.epa.gov/dashboard/DTXSID00357485 | |
Description | DSSTox provides a high quality public chemistry resource for supporting improved predictive toxicology. | |
Record name | 4,5-Dichlorophthalonitrile | |
Source | European Chemicals Agency (ECHA) | |
URL | https://echa.europa.eu/information-on-chemicals | |
Description | The European Chemicals Agency (ECHA) is an agency of the European Union which is the driving force among regulatory authorities in implementing the EU's groundbreaking chemicals legislation for the benefit of human health and the environment as well as for innovation and competitiveness. | |
Explanation | Use of the information, documents and data from the ECHA website is subject to the terms and conditions of this Legal Notice, and subject to other binding limitations provided for under applicable law, the information, documents and data made available on the ECHA website may be reproduced, distributed and/or used, totally or in part, for non-commercial purposes provided that ECHA is acknowledged as the source: "Source: European Chemicals Agency, http://echa.europa.eu/". Such acknowledgement must be included in each copy of the material. ECHA permits and encourages organisations and individuals to create links to the ECHA website under the following cumulative conditions: Links can only be made to webpages that provide a link to the Legal Notice page. | |
Retrosynthesis Analysis
AI-Powered Synthesis Planning: Our tool employs the Template_relevance Pistachio, Template_relevance Bkms_metabolic, Template_relevance Pistachio_ringbreaker, Template_relevance Reaxys, Template_relevance Reaxys_biocatalysis model, leveraging a vast database of chemical reactions to predict feasible synthetic routes.
One-Step Synthesis Focus: Specifically designed for one-step synthesis, it provides concise and direct routes for your target compounds, streamlining the synthesis process.
Accurate Predictions: Utilizing the extensive PISTACHIO, BKMS_METABOLIC, PISTACHIO_RINGBREAKER, REAXYS, REAXYS_BIOCATALYSIS database, our tool offers high-accuracy predictions, reflecting the latest in chemical research and data.
Strategy Settings
Precursor scoring | Relevance Heuristic |
---|---|
Min. plausibility | 0.01 |
Model | Template_relevance |
Template Set | Pistachio/Bkms_metabolic/Pistachio_ringbreaker/Reaxys/Reaxys_biocatalysis |
Top-N result to add to graph | 6 |
Feasible Synthetic Routes
Q & A
Q1: What is the primary use of 4,5-Dichlorophthalonitrile in current research?
A1: 4,5-Dichlorophthalonitrile serves as a key starting material for synthesizing phthalocyanines [, , , , , , , , , ]. These macrocyclic compounds exhibit interesting optical and electronic properties, making them suitable for various applications, including dyes, pigments, and potential components in organic electronic devices.
Q2: How are phthalocyanines synthesized using 4,5-Dichlorophthalonitrile?
A2: Phthalocyanines are typically synthesized through a cyclotetramerization reaction. This reaction involves four molecules of 4,5-Dichlorophthalonitrile reacting with a metal salt or in the presence of a base to form the macrocyclic phthalocyanine structure [, , , , , ].
Q3: Can the structure of phthalocyanines derived from 4,5-Dichlorophthalonitrile be modified?
A3: Yes, the structure of phthalocyanines can be readily modified by introducing various substituents onto the 4,5-Dichlorophthalonitrile scaffold before the cyclotetramerization reaction. This allows for fine-tuning of the phthalocyanine's properties, such as solubility, aggregation behavior, and electronic properties [, , , , , , , , ].
Q4: What types of substituents have been successfully incorporated into phthalocyanines using 4,5-Dichlorophthalonitrile?
A4: Researchers have successfully incorporated a wide range of substituents, including alkoxy groups, aryloxy groups, pyrazole groups, and even ferrocenylethynyl moieties, into phthalocyanines using 4,5-Dichlorophthalonitrile as a starting point [, , , , , , , ]. These modifications allow for the development of phthalocyanines with tailored properties.
Q5: Are there specific examples of how modifying 4,5-Dichlorophthalonitrile impacts the properties of the resulting phthalocyanines?
A5: Yes, for example, incorporating bulky tert-butyl groups into the phthalocyanine structure led to compounds with improved solubility and reduced aggregation in solution []. Furthermore, introducing electron-donating alkoxy groups and electron-withdrawing chloro substituents resulted in phthalocyanines with interesting electronic properties, potentially useful in nonlinear optical materials [].
Q6: Have hybrid phthalocyanine systems been developed using 4,5-Dichlorophthalonitrile?
A6: Yes, researchers have explored creating hybrid macrocycles by reacting 4,5-Dichlorophthalonitrile with 2,3-dicyanonaphthalene. These hybrids, termed X-RnBsub(Pcx-Ncy), have shown promising characteristics, such as broad absorption spectra, beneficial for light harvesting in organic solar cells [].
Q7: Can you elaborate on the "statistical" synthetic methodology used for synthesizing hybrid phthalocyanines?
A7: The "statistical" method involves reacting a mixture of 4,5-Dichlorophthalonitrile and 2,3-dicyanonaphthalene with boron trichloride. This approach yields a mixture of hybrid BsubPcs and BsubNcs with varying ratios of the two building blocks. By adjusting the reaction conditions, researchers can target specific hybrid compositions, such as X-RnBsub(Pc2-Nc1), which have demonstrated favorable properties for organic electronic applications [].
Q8: What analytical techniques are commonly employed to characterize 4,5-Dichlorophthalonitrile derivatives and the resulting phthalocyanines?
A8: Common techniques include nuclear magnetic resonance spectroscopy (NMR) for structural elucidation, infrared spectroscopy (IR) for identifying functional groups, mass spectrometry (MS) for determining molecular weight, and UV-Vis spectroscopy for studying their electronic properties [, , , , , , , , ].
Q9: Has computational chemistry played a role in understanding 4,5-Dichlorophthalonitrile-derived phthalocyanines?
A9: Yes, computational methods like density functional theory (DFT) are frequently employed to model the electronic structures, optical properties, and redox behavior of these phthalocyanine derivatives. This theoretical insight helps researchers design and optimize phthalocyanines for specific applications [, ].
Disclaimer and Information on In-Vitro Research Products
Please be aware that all articles and product information presented on BenchChem are intended solely for informational purposes. The products available for purchase on BenchChem are specifically designed for in-vitro studies, which are conducted outside of living organisms. In-vitro studies, derived from the Latin term "in glass," involve experiments performed in controlled laboratory settings using cells or tissues. It is important to note that these products are not categorized as medicines or drugs, and they have not received approval from the FDA for the prevention, treatment, or cure of any medical condition, ailment, or disease. We must emphasize that any form of bodily introduction of these products into humans or animals is strictly prohibited by law. It is essential to adhere to these guidelines to ensure compliance with legal and ethical standards in research and experimentation.