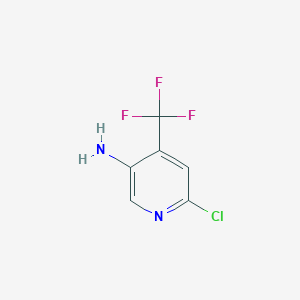
6-Chloro-4-(trifluoromethyl)pyridin-3-amine
Overview
Description
6-Chloro-4-(trifluoromethyl)pyridin-3-amine is a chemical compound characterized by the presence of a chloro group at the 6th position, a trifluoromethyl group at the 4th position, and an amine group at the 3rd position on a pyridine ring.
Biochemical Analysis
Biochemical Properties
6-Chloro-4-(trifluoromethyl)pyridin-3-amine plays a significant role in various biochemical reactions. It interacts with several enzymes, proteins, and other biomolecules. For instance, it has been observed to interact with enzymes involved in the trifluoromethylation process, which is crucial in the synthesis of pharmaceuticals and agrochemicals . The nature of these interactions often involves the formation of covalent bonds, leading to the modification of the enzyme’s active site and altering its activity.
Cellular Effects
The effects of this compound on various types of cells and cellular processes are profound. It influences cell function by modulating cell signaling pathways, gene expression, and cellular metabolism. For example, it has been shown to affect the expression of genes involved in the metabolic pathways of pyridine derivatives . Additionally, it can alter cellular metabolism by inhibiting or activating specific enzymes, leading to changes in the levels of metabolites within the cell.
Molecular Mechanism
At the molecular level, this compound exerts its effects through several mechanisms. It binds to specific biomolecules, leading to enzyme inhibition or activation. For instance, it can inhibit enzymes involved in the trifluoromethylation process by binding to their active sites . This binding interaction can result in changes in gene expression, further influencing cellular function and metabolism.
Temporal Effects in Laboratory Settings
In laboratory settings, the effects of this compound can change over time. The compound’s stability and degradation are critical factors that influence its long-term effects on cellular function. Studies have shown that this compound is relatively stable under standard laboratory conditions, but it can degrade over time, leading to a decrease in its efficacy . Long-term exposure to the compound in in vitro or in vivo studies has shown that it can have lasting effects on cellular function, including alterations in gene expression and metabolic pathways.
Dosage Effects in Animal Models
The effects of this compound vary with different dosages in animal models. At lower doses, the compound may have minimal effects, while higher doses can lead to significant changes in cellular function and metabolism. Threshold effects have been observed, where a certain dosage is required to elicit a noticeable response . Additionally, high doses of this compound can result in toxic or adverse effects, including cellular damage and disruption of normal metabolic processes.
Metabolic Pathways
This compound is involved in several metabolic pathways, interacting with various enzymes and cofactors. It can influence metabolic flux and metabolite levels by modulating the activity of enzymes involved in the metabolism of pyridine derivatives . These interactions can lead to changes in the levels of specific metabolites, affecting overall cellular metabolism.
Transport and Distribution
The transport and distribution of this compound within cells and tissues are mediated by specific transporters and binding proteins. These interactions can influence the localization and accumulation of the compound within different cellular compartments . Understanding the transport and distribution mechanisms is crucial for determining the compound’s efficacy and potential side effects.
Subcellular Localization
The subcellular localization of this compound plays a significant role in its activity and function. The compound can be directed to specific compartments or organelles within the cell through targeting signals or post-translational modifications . These localization mechanisms can influence the compound’s interactions with biomolecules and its overall biochemical effects.
Preparation Methods
Synthetic Routes and Reaction Conditions: The synthesis of 6-Chloro-4-(trifluoromethyl)pyridin-3-amine typically involves the introduction of the chloro and trifluoromethyl groups onto a pyridine ring, followed by the introduction of the amine group. One common method involves the chlorination of 4-(trifluoromethyl)pyridine, followed by amination at the 3rd position. The reaction conditions often include the use of chlorinating agents such as thionyl chloride or phosphorus pentachloride, and amination can be achieved using ammonia or amine derivatives under controlled temperatures and pressures .
Industrial Production Methods: Industrial production of this compound may involve large-scale chlorination and amination processes, utilizing continuous flow reactors to ensure consistent quality and yield. The use of catalysts and optimized reaction conditions can enhance the efficiency of the production process .
Chemical Reactions Analysis
Types of Reactions: 6-Chloro-4-(trifluoromethyl)pyridin-3-amine undergoes various chemical reactions, including:
Substitution Reactions: The chloro group can be substituted with other nucleophiles, such as hydroxyl or alkoxy groups, under appropriate conditions.
Oxidation and Reduction Reactions: The compound can be oxidized or reduced to form different derivatives, depending on the reagents and conditions used.
Coupling Reactions: It can participate in Suzuki-Miyaura coupling reactions to form carbon-carbon bonds with other aromatic compounds.
Common Reagents and Conditions:
Substitution Reactions: Reagents such as sodium hydroxide or alkoxides in polar solvents.
Oxidation Reactions: Oxidizing agents like potassium permanganate or hydrogen peroxide.
Reduction Reactions: Reducing agents such as lithium aluminum hydride or sodium borohydride.
Coupling Reactions: Palladium catalysts and boronic acids under mild conditions.
Major Products Formed: The major products formed from these reactions include various substituted pyridines, amine derivatives, and coupled aromatic compounds .
Scientific Research Applications
6-Chloro-4-(trifluoromethyl)pyridin-3-amine has a wide range of applications in scientific research:
Mechanism of Action
The mechanism of action of 6-Chloro-4-(trifluoromethyl)pyridin-3-amine involves its interaction with specific molecular targets, such as enzymes or receptors. The trifluoromethyl group enhances the compound’s lipophilicity, allowing it to easily penetrate cell membranes and interact with intracellular targets. The chloro and amine groups contribute to the compound’s binding affinity and specificity towards its targets, leading to various biological effects .
Comparison with Similar Compounds
- 2-Chloro-4-(trifluoromethyl)pyridine
- 4-(Trifluoromethyl)pyridine
- 4,4’-bis(trifluoromethyl)-2,2’-bipyridine
Comparison: 6-Chloro-4-(trifluoromethyl)pyridin-3-amine is unique due to the specific positioning of the chloro, trifluoromethyl, and amine groups on the pyridine ring. This unique arrangement imparts distinct chemical and biological properties, making it a valuable compound for various applications. In comparison, similar compounds may lack one or more of these functional groups, resulting in different reactivity and applications .
Biological Activity
6-Chloro-4-(trifluoromethyl)pyridin-3-amine is a heterocyclic compound that has garnered attention due to its diverse biological activities and potential applications in medicinal chemistry and agriculture. This article explores its biological activity, synthesis, and relevant research findings, supported by data tables and case studies.
Chemical Structure and Properties
The compound features a pyridine ring substituted with a chlorine atom at the 6-position and a trifluoromethyl group at the 4-position. Its molecular formula is , with a molecular weight of approximately 196.56 g/mol. The presence of the trifluoromethyl group enhances its lipophilicity and metabolic stability, which are critical for its biological activity.
Biological Activity Overview
Research indicates that this compound exhibits promising biological activities, particularly in the following areas:
- Antimicrobial Activity : Compounds similar to this compound have shown antibacterial and antifungal properties. The trifluoromethyl group is known to enhance the efficacy of such compounds against various pathogens .
- Antichlamydial Activity : Studies have demonstrated that derivatives containing the trifluoromethyl group can inhibit the growth of Chlamydia trachomatis, suggesting potential for developing selective treatments against this pathogen .
- Pharmacological Applications : The compound has been investigated for its interactions with specific enzymes and receptors, indicating its potential as a lead compound in drug development.
Synthesis Methods
The synthesis of this compound can be achieved through several methods, including:
- Direct Halogenation : Chlorination of 4-(trifluoromethyl)pyridine derivatives.
- Nucleophilic Substitution : Reaction of pyridine derivatives with suitable electrophiles.
- Acylation Reactions : The amine group can react with acyl chlorides to yield various acylated products.
Antibacterial Activity
A study evaluated the antibacterial activity of several pyridine derivatives, including those similar to this compound. The minimum inhibitory concentration (MIC) values against Staphylococcus aureus were found to be between 3.12 and 12.5 μg/mL, demonstrating significant efficacy compared to standard antibiotics like ciprofloxacin (2 μg/mL) .
Antichlamydial Selectivity
Research on antichlamydial compounds indicated that derivatives containing the trifluoromethyl group exhibited selective activity against Chlamydia trachomatis. One particular derivative showed an IC50 value of 5.2 μg/mL, outperforming several established treatments in terms of selectivity and efficacy without affecting host cell viability .
Comparative Analysis with Similar Compounds
To better understand the biological activity of this compound, a comparative analysis with structurally similar compounds is presented below:
Compound Name | CAS Number | Key Features | MIC (μg/mL) |
---|---|---|---|
2-Chloro-6-(trifluoromethyl)pyridin-3-amine | 117519-09-2 | Similar trifluoromethyl substitution; different Cl position | 10 |
3-Amino-2-chloro-6-(trifluoromethyl)pyridine | 1211590-44-1 | Contains amino group; different reactivity | 8 |
4-Chloro-6-(trifluoromethyl)pyridin-3-amines | 166770-70-3 | Similar halogenated structure; varied biological activity | 15 |
Properties
IUPAC Name |
6-chloro-4-(trifluoromethyl)pyridin-3-amine | |
---|---|---|
Source | PubChem | |
URL | https://pubchem.ncbi.nlm.nih.gov | |
Description | Data deposited in or computed by PubChem | |
InChI |
InChI=1S/C6H4ClF3N2/c7-5-1-3(6(8,9)10)4(11)2-12-5/h1-2H,11H2 | |
Source | PubChem | |
URL | https://pubchem.ncbi.nlm.nih.gov | |
Description | Data deposited in or computed by PubChem | |
InChI Key |
YAQCRBPWLAGKAC-UHFFFAOYSA-N | |
Source | PubChem | |
URL | https://pubchem.ncbi.nlm.nih.gov | |
Description | Data deposited in or computed by PubChem | |
Canonical SMILES |
C1=C(C(=CN=C1Cl)N)C(F)(F)F | |
Source | PubChem | |
URL | https://pubchem.ncbi.nlm.nih.gov | |
Description | Data deposited in or computed by PubChem | |
Molecular Formula |
C6H4ClF3N2 | |
Source | PubChem | |
URL | https://pubchem.ncbi.nlm.nih.gov | |
Description | Data deposited in or computed by PubChem | |
Molecular Weight |
196.56 g/mol | |
Source | PubChem | |
URL | https://pubchem.ncbi.nlm.nih.gov | |
Description | Data deposited in or computed by PubChem | |
Retrosynthesis Analysis
AI-Powered Synthesis Planning: Our tool employs the Template_relevance Pistachio, Template_relevance Bkms_metabolic, Template_relevance Pistachio_ringbreaker, Template_relevance Reaxys, Template_relevance Reaxys_biocatalysis model, leveraging a vast database of chemical reactions to predict feasible synthetic routes.
One-Step Synthesis Focus: Specifically designed for one-step synthesis, it provides concise and direct routes for your target compounds, streamlining the synthesis process.
Accurate Predictions: Utilizing the extensive PISTACHIO, BKMS_METABOLIC, PISTACHIO_RINGBREAKER, REAXYS, REAXYS_BIOCATALYSIS database, our tool offers high-accuracy predictions, reflecting the latest in chemical research and data.
Strategy Settings
Precursor scoring | Relevance Heuristic |
---|---|
Min. plausibility | 0.01 |
Model | Template_relevance |
Template Set | Pistachio/Bkms_metabolic/Pistachio_ringbreaker/Reaxys/Reaxys_biocatalysis |
Top-N result to add to graph | 6 |
Feasible Synthetic Routes
Disclaimer and Information on In-Vitro Research Products
Please be aware that all articles and product information presented on BenchChem are intended solely for informational purposes. The products available for purchase on BenchChem are specifically designed for in-vitro studies, which are conducted outside of living organisms. In-vitro studies, derived from the Latin term "in glass," involve experiments performed in controlled laboratory settings using cells or tissues. It is important to note that these products are not categorized as medicines or drugs, and they have not received approval from the FDA for the prevention, treatment, or cure of any medical condition, ailment, or disease. We must emphasize that any form of bodily introduction of these products into humans or animals is strictly prohibited by law. It is essential to adhere to these guidelines to ensure compliance with legal and ethical standards in research and experimentation.