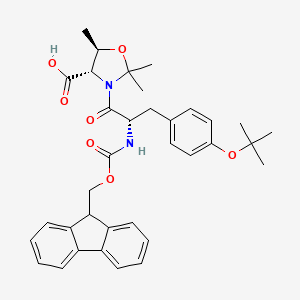
Fmoc-Tyr(tBu)-Thr(Psi(Me,Me)pro)-OH
Overview
Description
Fmoc-Tyr(tBu)-Thr(Psi(Me,Me)pro)-OH is a synthetic peptide derivative used in various biochemical and pharmaceutical research applications. The compound is characterized by the presence of a fluorenylmethyloxycarbonyl (Fmoc) protecting group, a tert-butyl (tBu) group, and a unique Psi(Me,Me)pro moiety. These modifications enhance the stability and solubility of the peptide, making it suitable for use in solid-phase peptide synthesis and other research applications.
Preparation Methods
Synthetic Routes and Reaction Conditions
The synthesis of Fmoc-Tyr(tBu)-Thr(Psi(Me,Me)pro)-OH typically involves solid-phase peptide synthesis (SPPS) using Fmoc chemistry. The process begins with the attachment of the first amino acid to a solid resin, followed by sequential addition of protected amino acids. The Fmoc group is removed using piperidine, and the peptide chain is elongated by coupling reactions with the next amino acid .
Industrial Production Methods
In industrial settings, the production of this compound follows similar SPPS protocols but on a larger scale. Automated peptide synthesizers are often employed to ensure high efficiency and reproducibility. The use of green solvents and optimized reaction conditions can minimize environmental impact and improve the overall yield .
Chemical Reactions Analysis
Types of Reactions
Fmoc-Tyr(tBu)-Thr(Psi(Me,Me)pro)-OH can undergo various chemical reactions, including:
Deprotection: Removal of the Fmoc group using piperidine.
Coupling: Formation of peptide bonds with other amino acids.
Oxidation and Reduction: Modifications of side chains or functional groups.
Common Reagents and Conditions
Piperidine: Used for Fmoc deprotection.
Carbodiimides (e.g., DIC, EDC): Used for coupling reactions.
Oxidizing agents (e.g., hydrogen peroxide): Used for oxidation reactions.
Major Products Formed
The primary product formed from these reactions is the elongated peptide chain with specific modifications at the tyrosine and threonine residues. The final product, this compound, is obtained after cleavage from the resin and purification .
Scientific Research Applications
Chemistry
Fmoc-Tyr(tBu)-Thr(Psi(Me,Me)pro)-OH is widely used in peptide synthesis and combinatorial chemistry. Its stability and solubility make it an ideal building block for creating complex peptide libraries .
Biology
In biological research, this compound is used to study protein-protein interactions, enzyme-substrate specificity, and receptor-ligand binding. It serves as a model peptide for investigating the structural and functional properties of proteins .
Medicine
It can be used to design peptide-based therapeutics and diagnostic agents .
Industry
In the industrial sector, this compound is used in the production of peptide-based materials, such as hydrogels and nanomaterials, for various applications, including drug delivery and tissue engineering .
Mechanism of Action
The mechanism of action of Fmoc-Tyr(tBu)-Thr(Psi(Me,Me)pro)-OH involves its ability to form stable peptide bonds and interact with specific molecular targets. The Fmoc group provides protection during synthesis, while the tBu and Psi(Me,Me)pro groups enhance the stability and solubility of the peptide. These modifications allow the compound to interact with enzymes, receptors, and other biomolecules, facilitating various biochemical and pharmacological processes .
Comparison with Similar Compounds
Similar Compounds
- Fmoc-Tyr(tBu)-Ser(Psi(Me,Me)pro)-OH
- Fmoc-Tyr(tBu)-Thr(tBu)-OH
- Fmoc-Tyr(tBu)-Thr(OH)-OH
Uniqueness
Fmoc-Tyr(tBu)-Thr(Psi(Me,Me)pro)-OH is unique due to the presence of the Psi(Me,Me)pro moiety, which provides enhanced stability and solubility compared to other similar compounds. This makes it particularly useful in applications requiring high stability and solubility, such as in drug development and peptide-based material production .
Properties
IUPAC Name |
(4S,5R)-3-[(2S)-2-(9H-fluoren-9-ylmethoxycarbonylamino)-3-[4-[(2-methylpropan-2-yl)oxy]phenyl]propanoyl]-2,2,5-trimethyl-1,3-oxazolidine-4-carboxylic acid | |
---|---|---|
Source | PubChem | |
URL | https://pubchem.ncbi.nlm.nih.gov | |
Description | Data deposited in or computed by PubChem | |
InChI |
InChI=1S/C35H40N2O7/c1-21-30(32(39)40)37(35(5,6)43-21)31(38)29(19-22-15-17-23(18-16-22)44-34(2,3)4)36-33(41)42-20-28-26-13-9-7-11-24(26)25-12-8-10-14-27(25)28/h7-18,21,28-30H,19-20H2,1-6H3,(H,36,41)(H,39,40)/t21-,29+,30+/m1/s1 | |
Source | PubChem | |
URL | https://pubchem.ncbi.nlm.nih.gov | |
Description | Data deposited in or computed by PubChem | |
InChI Key |
JYSMURJYNLWZNY-RIGQTMPJSA-N | |
Source | PubChem | |
URL | https://pubchem.ncbi.nlm.nih.gov | |
Description | Data deposited in or computed by PubChem | |
Canonical SMILES |
CC1C(N(C(O1)(C)C)C(=O)C(CC2=CC=C(C=C2)OC(C)(C)C)NC(=O)OCC3C4=CC=CC=C4C5=CC=CC=C35)C(=O)O | |
Source | PubChem | |
URL | https://pubchem.ncbi.nlm.nih.gov | |
Description | Data deposited in or computed by PubChem | |
Isomeric SMILES |
C[C@@H]1[C@H](N(C(O1)(C)C)C(=O)[C@H](CC2=CC=C(C=C2)OC(C)(C)C)NC(=O)OCC3C4=CC=CC=C4C5=CC=CC=C35)C(=O)O | |
Source | PubChem | |
URL | https://pubchem.ncbi.nlm.nih.gov | |
Description | Data deposited in or computed by PubChem | |
Molecular Formula |
C35H40N2O7 | |
Source | PubChem | |
URL | https://pubchem.ncbi.nlm.nih.gov | |
Description | Data deposited in or computed by PubChem | |
Molecular Weight |
600.7 g/mol | |
Source | PubChem | |
URL | https://pubchem.ncbi.nlm.nih.gov | |
Description | Data deposited in or computed by PubChem | |
Retrosynthesis Analysis
AI-Powered Synthesis Planning: Our tool employs the Template_relevance Pistachio, Template_relevance Bkms_metabolic, Template_relevance Pistachio_ringbreaker, Template_relevance Reaxys, Template_relevance Reaxys_biocatalysis model, leveraging a vast database of chemical reactions to predict feasible synthetic routes.
One-Step Synthesis Focus: Specifically designed for one-step synthesis, it provides concise and direct routes for your target compounds, streamlining the synthesis process.
Accurate Predictions: Utilizing the extensive PISTACHIO, BKMS_METABOLIC, PISTACHIO_RINGBREAKER, REAXYS, REAXYS_BIOCATALYSIS database, our tool offers high-accuracy predictions, reflecting the latest in chemical research and data.
Strategy Settings
Precursor scoring | Relevance Heuristic |
---|---|
Min. plausibility | 0.01 |
Model | Template_relevance |
Template Set | Pistachio/Bkms_metabolic/Pistachio_ringbreaker/Reaxys/Reaxys_biocatalysis |
Top-N result to add to graph | 6 |
Feasible Synthetic Routes
Disclaimer and Information on In-Vitro Research Products
Please be aware that all articles and product information presented on BenchChem are intended solely for informational purposes. The products available for purchase on BenchChem are specifically designed for in-vitro studies, which are conducted outside of living organisms. In-vitro studies, derived from the Latin term "in glass," involve experiments performed in controlled laboratory settings using cells or tissues. It is important to note that these products are not categorized as medicines or drugs, and they have not received approval from the FDA for the prevention, treatment, or cure of any medical condition, ailment, or disease. We must emphasize that any form of bodily introduction of these products into humans or animals is strictly prohibited by law. It is essential to adhere to these guidelines to ensure compliance with legal and ethical standards in research and experimentation.