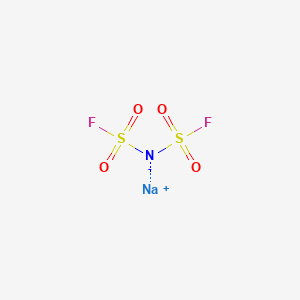
Sodium bis(fluorosulfonyl)imide
Overview
Description
Sodium bis(fluorosulfonyl)imide (Sodium bis(fluorosulfonyl)amide) is a chemical compound with the molecular formula NaFSI. It is a colorless liquid with a melting point of 17°C and a boiling point of 170°C. This compound is known for its high thermal stability and excellent solubility in water. This compound is widely used in various scientific research applications, including chemistry, biology, medicine, and industry.
Mechanism of Action
Target of Action
Sodium bis(fluorosulfonyl)imide primarily targets electrochemical storage devices, such as lithium-ion batteries . It serves as an electrolyte in these devices, facilitating the movement of ions between the anode and cathode during the charge and discharge cycles .
Mode of Action
this compound interacts with its targets by providing a medium for ion transport. It enhances the performance of lithium-ion batteries by improving the lithium-ion transport, especially when high current densities are applied . This compound outperforms commonly used aprotic ionic liquids, maintaining the advantages and safety of ionic liquid-based electrolytes .
Biochemical Pathways
It facilitates the flow of ions between the anode and cathode, which is essential for the charge and discharge processes in lithium-ion batteries .
Pharmacokinetics
Its properties such as solubility, stability, and conductivity are critical for its function as an electrolyte in batteries .
Result of Action
The use of this compound as an electrolyte results in improved performance of lithium-ion batteries. It enhances the safety of these batteries by reducing flammability and volatility, which are common issues with traditional organic carbonate-based electrolytes .
Action Environment
The action of this compound is influenced by environmental factors such as temperature and humidity. It should be stored under an inert atmosphere at room temperature . It is also hygroscopic, meaning it can absorb moisture from the environment, which could potentially affect its performance .
Biochemical Analysis
Biochemical Properties
Sodium bis(fluorosulfonyl)imide plays a significant role in biochemical reactions, particularly in the context of its interactions with enzymes, proteins, and other biomolecules. It has been observed to interact with sodium metal anodes, enabling their use in room-temperature sodium metal batteries . The nature of these interactions involves the formation of stable complexes that facilitate the efficient transfer of sodium ions.
Cellular Effects
The effects of this compound on various types of cells and cellular processes are profound. It influences cell function by affecting cell signaling pathways, gene expression, and cellular metabolism. Studies have shown that this compound can enhance the electrochemical activity of cells, leading to improved performance in applications such as sodium metal batteries .
Molecular Mechanism
At the molecular level, this compound exerts its effects through specific binding interactions with biomolecules. It has been identified as a “magic anion” for electrochemistry due to its ability to form stable complexes with sodium ions . This stability is crucial for the efficient functioning of sodium metal batteries, as it prevents the degradation of the electrolyte and ensures consistent performance.
Temporal Effects in Laboratory Settings
In laboratory settings, the effects of this compound change over time. The compound exhibits high stability, with minimal degradation observed over extended periods . Long-term studies have shown that this compound maintains its efficacy in enhancing cellular function, making it a reliable component in biochemical applications.
Dosage Effects in Animal Models
The effects of this compound vary with different dosages in animal models. At lower doses, the compound has been found to be non-toxic and effective in enhancing cellular function. At higher doses, it may exhibit toxic effects, including potential damage to cellular structures . It is crucial to determine the optimal dosage to maximize benefits while minimizing adverse effects.
Metabolic Pathways
This compound is involved in specific metabolic pathways, interacting with enzymes and cofactors that facilitate its function. The compound influences metabolic flux and metabolite levels, contributing to its role in enhancing cellular function . Understanding these pathways is essential for optimizing its use in biochemical applications.
Transport and Distribution
Within cells and tissues, this compound is transported and distributed through interactions with specific transporters and binding proteins. These interactions ensure its proper localization and accumulation, which are critical for its function . The compound’s distribution patterns are essential for its efficacy in various applications.
Subcellular Localization
This compound exhibits specific subcellular localization, which affects its activity and function. Targeting signals and post-translational modifications direct the compound to specific compartments or organelles, ensuring its optimal performance . Understanding these localization mechanisms is vital for harnessing its full potential in biochemical applications.
Preparation Methods
Synthetic Routes and Reaction Conditions: Sodium bis(fluorosulfonyl)imide can be synthesized through the reaction of sodium fluoride (NaF) with fluorosulfonic acid (HSO3F). The reaction is typically carried out under controlled conditions to ensure the formation of the desired product.
Industrial Production Methods: In industrial settings, the production of this compound involves large-scale reactions with stringent quality control measures to ensure the purity and consistency of the compound. The compound is produced in powder or crystalline form and is often used as an electrolyte in sodium-ion batteries due to its excellent performance and low cost.
Chemical Reactions Analysis
Types of Reactions: Sodium bis(fluorosulfonyl)imide undergoes various types of chemical reactions, including oxidation, reduction, and substitution reactions.
Common Reagents and Conditions:
Oxidation Reactions: this compound can act as an oxidizing agent in the presence of reducing agents.
Reduction Reactions: It can be reduced using suitable reducing agents under controlled conditions.
Substitution Reactions: The compound can undergo nucleophilic substitution reactions with various nucleophiles.
Major Products Formed: The major products formed from these reactions include various sodium salts, fluorosulfonic acid derivatives, and other related compounds.
Scientific Research Applications
Sodium bis(fluorosulfonyl)imide is extensively used in scientific research due to its unique properties and versatility. It is commonly employed as an electrolyte in sodium-ion batteries, where it offers excellent performance and stability. Additionally, it is used in organic synthesis, as a reagent in chemical reactions, and in the development of new materials and compounds.
Comparison with Similar Compounds
Sodium hexafluorophosphate (NaPF6)
Sodium trifluoromethanesulfonate (NaTf)
Sodium bis(trifluoromethanesulfonyl)imide (NaTFSI)
Properties
IUPAC Name |
sodium;bis(fluorosulfonyl)azanide | |
---|---|---|
Source | PubChem | |
URL | https://pubchem.ncbi.nlm.nih.gov | |
Description | Data deposited in or computed by PubChem | |
InChI |
InChI=1S/F2NO4S2.Na/c1-8(4,5)3-9(2,6)7;/q-1;+1 | |
Source | PubChem | |
URL | https://pubchem.ncbi.nlm.nih.gov | |
Description | Data deposited in or computed by PubChem | |
InChI Key |
VCCATSJUUVERFU-UHFFFAOYSA-N | |
Source | PubChem | |
URL | https://pubchem.ncbi.nlm.nih.gov | |
Description | Data deposited in or computed by PubChem | |
Canonical SMILES |
[N-](S(=O)(=O)F)S(=O)(=O)F.[Na+] | |
Source | PubChem | |
URL | https://pubchem.ncbi.nlm.nih.gov | |
Description | Data deposited in or computed by PubChem | |
Molecular Formula |
F2NNaO4S2 | |
Source | PubChem | |
URL | https://pubchem.ncbi.nlm.nih.gov | |
Description | Data deposited in or computed by PubChem | |
Molecular Weight |
203.13 g/mol | |
Source | PubChem | |
URL | https://pubchem.ncbi.nlm.nih.gov | |
Description | Data deposited in or computed by PubChem | |
CAS No. |
100669-96-3 | |
Record name | Sodium Bis(fluorosulfonyl)imide | |
Source | European Chemicals Agency (ECHA) | |
URL | https://echa.europa.eu/information-on-chemicals | |
Description | The European Chemicals Agency (ECHA) is an agency of the European Union which is the driving force among regulatory authorities in implementing the EU's groundbreaking chemicals legislation for the benefit of human health and the environment as well as for innovation and competitiveness. | |
Explanation | Use of the information, documents and data from the ECHA website is subject to the terms and conditions of this Legal Notice, and subject to other binding limitations provided for under applicable law, the information, documents and data made available on the ECHA website may be reproduced, distributed and/or used, totally or in part, for non-commercial purposes provided that ECHA is acknowledged as the source: "Source: European Chemicals Agency, http://echa.europa.eu/". Such acknowledgement must be included in each copy of the material. ECHA permits and encourages organisations and individuals to create links to the ECHA website under the following cumulative conditions: Links can only be made to webpages that provide a link to the Legal Notice page. | |
Retrosynthesis Analysis
AI-Powered Synthesis Planning: Our tool employs the Template_relevance Pistachio, Template_relevance Bkms_metabolic, Template_relevance Pistachio_ringbreaker, Template_relevance Reaxys, Template_relevance Reaxys_biocatalysis model, leveraging a vast database of chemical reactions to predict feasible synthetic routes.
One-Step Synthesis Focus: Specifically designed for one-step synthesis, it provides concise and direct routes for your target compounds, streamlining the synthesis process.
Accurate Predictions: Utilizing the extensive PISTACHIO, BKMS_METABOLIC, PISTACHIO_RINGBREAKER, REAXYS, REAXYS_BIOCATALYSIS database, our tool offers high-accuracy predictions, reflecting the latest in chemical research and data.
Strategy Settings
Precursor scoring | Relevance Heuristic |
---|---|
Min. plausibility | 0.01 |
Model | Template_relevance |
Template Set | Pistachio/Bkms_metabolic/Pistachio_ringbreaker/Reaxys/Reaxys_biocatalysis |
Top-N result to add to graph | 6 |
Feasible Synthetic Routes
Q1: How does NaFSI improve the performance of sodium-ion batteries?
A1: NaFSI offers several advantages as an electrolyte component in SIBs:
- High ionic conductivity: NaFSI-based electrolytes exhibit high ionic conductivity, even at low temperatures, facilitating efficient ion transport and enhancing battery performance. [, , , ]
- Stable Solid Electrolyte Interphase (SEI) Formation: NaFSI contributes to the formation of a stable and ion-conductive SEI layer on both anode and cathode surfaces. This protective layer prevents further unwanted reactions between the electrode and electrolyte, improving battery lifespan. [, , , ]
- Wide Electrochemical Window: NaFSI-based electrolytes demonstrate a wide electrochemical stability window, allowing for the use of high-voltage cathodes and increasing overall battery energy density. [, , ]
- Suppression of Dendrite Formation: NaFSI, particularly in high-concentration electrolytes, helps suppress dendrite formation on sodium metal anodes, enhancing safety and cycle life. [, , ]
Q2: How does NaFSI interact with the anode and cathode materials in SIBs?
A2: NaFSI decomposes during the initial battery cycles, forming a protective SEI layer on both the anode and cathode surfaces. This layer comprises inorganic components like NaF and organic species derived from electrolyte solvents. The specific composition and properties of the SEI layer depend on the electrolyte formulation and operating conditions. [, , , ]
Q3: What is the role of NaFSI concentration in the electrolyte?
A3: Electrolyte concentration significantly impacts NaFSI's behavior and battery performance:
- Dilute Electrolytes: In dilute electrolytes, NaFSI primarily exists as solvent-separated ion pairs, leading to good ionic conductivity but potentially weaker SEI formation. []
- Localized High-Concentration Electrolytes (LHCE): LHCEs utilize diluents to reduce overall salt concentration while maintaining the benefits of concentrated systems. This approach balances conductivity, viscosity, and cost. [, ]
Q4: What is the molecular formula, weight, and spectroscopic data for NaFSI?
A4:* Molecular Formula: NaFSI * IUPAC Name: Sodium imidodi(sulfonyl fluoride)* Molecular Weight: 210.04 g/mol* Spectroscopic Data: Characterized using techniques like FT-Raman and NMR (¹³C, ¹⁷O, ²³Na) to study solvation structures and interactions with solvents. []
Q5: What are the compatibility challenges of NaFSI with different battery components?
A5: NaFSI can be corrosive towards aluminum, a common current collector material, particularly at high voltages. Strategies to address this include protective coatings, alternative current collectors, and electrolyte additives that passivate aluminum surfaces. [, ]
Q6: How is computational chemistry used to study NaFSI-based electrolytes?
A6:
- Molecular Dynamics (MD) Simulations: MD simulations provide insights into the solvation structure of NaFSI in various solvents, ion transport mechanisms, and interactions with electrode surfaces. [, ]
- Ab Initio Calculations: These calculations contribute to a deeper understanding of electronic structure, bonding, and decomposition pathways of NaFSI, aiding in electrolyte design and optimization. []
Q7: What are the future research directions for NaFSI in sodium-ion batteries?
A7: Future research focuses on:
- Optimizing Electrolyte Formulations: Exploring novel solvent blends, additives, and salt concentrations to further enhance conductivity, stability, and safety. [, ]
- Understanding SEI Formation Mechanisms: In-depth characterization of the SEI layer using advanced techniques to tailor its properties for improved performance. [, ]
- Developing Sustainable Practices: Investigating alternative synthesis routes for NaFSI and exploring recycling strategies to minimize environmental impact. [, ]
- Expanding Applications: Evaluating NaFSI-based electrolytes in next-generation battery technologies beyond conventional SIBs. [, ]
Disclaimer and Information on In-Vitro Research Products
Please be aware that all articles and product information presented on BenchChem are intended solely for informational purposes. The products available for purchase on BenchChem are specifically designed for in-vitro studies, which are conducted outside of living organisms. In-vitro studies, derived from the Latin term "in glass," involve experiments performed in controlled laboratory settings using cells or tissues. It is important to note that these products are not categorized as medicines or drugs, and they have not received approval from the FDA for the prevention, treatment, or cure of any medical condition, ailment, or disease. We must emphasize that any form of bodily introduction of these products into humans or animals is strictly prohibited by law. It is essential to adhere to these guidelines to ensure compliance with legal and ethical standards in research and experimentation.