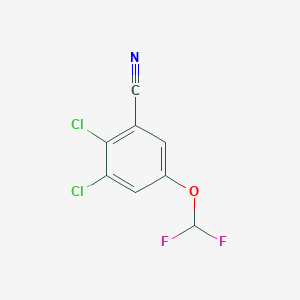
2,3-Dichloro-5-(difluoromethoxy)benzonitrile
Overview
Description
2,3-Dichloro-5-(difluoromethoxy)benzonitrile is a chemical compound known for its application as a pre-emergent herbicide. It belongs to the group of benzamide chemicals and is primarily used to control broadleaf and annual grass weeds in various crops such as corn, sunflowers, sorghum, and peanuts. This compound is also known by the name Diflufenican.
Preparation Methods
Synthetic Routes and Reaction Conditions
The synthesis of 2,3-Dichloro-5-(difluoromethoxy)benzonitrile involves several steps. One common method includes the reaction of 2,3-dichlorobenzonitrile with difluoromethoxy reagents under specific conditions. The reaction typically requires a catalyst and controlled temperature to ensure the desired product is obtained with high purity .
Industrial Production Methods
In industrial settings, the production of this compound is scaled up using optimized reaction conditions to maximize yield and minimize by-products. The process involves continuous monitoring and adjustment of reaction parameters such as temperature, pressure, and reagent concentrations to achieve efficient production .
Chemical Reactions Analysis
Types of Reactions
2,3-Dichloro-5-(difluoromethoxy)benzonitrile undergoes various chemical reactions, including:
Substitution Reactions: The compound can participate in nucleophilic substitution reactions where the chlorine atoms are replaced by other nucleophiles.
Oxidation and Reduction: It can undergo oxidation to form different oxidation states, although this is less common.
Hydrolysis: The compound can be hydrolyzed under acidic or basic conditions to yield corresponding acids or alcohols.
Common Reagents and Conditions
Common reagents used in these reactions include strong nucleophiles like sodium hydroxide for substitution reactions, oxidizing agents like potassium permanganate for oxidation, and acids or bases for hydrolysis .
Major Products Formed
The major products formed from these reactions depend on the specific conditions and reagents used. For example, substitution reactions can yield a variety of substituted benzonitriles, while hydrolysis can produce difluoromethoxybenzoic acid .
Scientific Research Applications
2,3-Dichloro-5-(difluoromethoxy)benzonitrile has several applications in scientific research:
Chemistry: It is used as an intermediate in the synthesis of other complex organic compounds.
Biology: The compound is studied for its effects on plant physiology and its potential use in controlling invasive plant species.
Medicine: Research is ongoing to explore its potential as a lead compound for developing new pharmaceuticals.
Industry: It is widely used in the agricultural industry as a herbicide to protect crops from weeds.
Mechanism of Action
The mechanism of action of 2,3-Dichloro-5-(difluoromethoxy)benzonitrile involves inhibiting specific enzymes in plants that are crucial for their growth. This inhibition leads to the disruption of essential metabolic pathways, ultimately causing the death of the targeted weeds. The molecular targets include enzymes involved in photosynthesis and cell division.
Comparison with Similar Compounds
Similar Compounds
Some compounds similar to 2,3-Dichloro-5-(difluoromethoxy)benzonitrile include:
2,3-Dichloro-5-(trifluoromethyl)pyridine: Another herbicide with similar applications but different chemical properties.
2,3-Dichloro-5-(trifluoromethoxy)benzonitrile: A closely related compound with slight variations in its chemical structure and reactivity.
Uniqueness
What sets this compound apart is its specific combination of chlorine and difluoromethoxy groups, which confer unique properties such as high efficacy in weed control and lower toxicity to non-target organisms. This makes it a preferred choice in agricultural applications.
Properties
IUPAC Name |
2,3-dichloro-5-(difluoromethoxy)benzonitrile | |
---|---|---|
Source | PubChem | |
URL | https://pubchem.ncbi.nlm.nih.gov | |
Description | Data deposited in or computed by PubChem | |
InChI |
InChI=1S/C8H3Cl2F2NO/c9-6-2-5(14-8(11)12)1-4(3-13)7(6)10/h1-2,8H | |
Source | PubChem | |
URL | https://pubchem.ncbi.nlm.nih.gov | |
Description | Data deposited in or computed by PubChem | |
InChI Key |
DLYYDDSOKMJZTC-UHFFFAOYSA-N | |
Source | PubChem | |
URL | https://pubchem.ncbi.nlm.nih.gov | |
Description | Data deposited in or computed by PubChem | |
Canonical SMILES |
C1=C(C=C(C(=C1C#N)Cl)Cl)OC(F)F | |
Source | PubChem | |
URL | https://pubchem.ncbi.nlm.nih.gov | |
Description | Data deposited in or computed by PubChem | |
Molecular Formula |
C8H3Cl2F2NO | |
Source | PubChem | |
URL | https://pubchem.ncbi.nlm.nih.gov | |
Description | Data deposited in or computed by PubChem | |
Molecular Weight |
238.01 g/mol | |
Source | PubChem | |
URL | https://pubchem.ncbi.nlm.nih.gov | |
Description | Data deposited in or computed by PubChem | |
Retrosynthesis Analysis
AI-Powered Synthesis Planning: Our tool employs the Template_relevance Pistachio, Template_relevance Bkms_metabolic, Template_relevance Pistachio_ringbreaker, Template_relevance Reaxys, Template_relevance Reaxys_biocatalysis model, leveraging a vast database of chemical reactions to predict feasible synthetic routes.
One-Step Synthesis Focus: Specifically designed for one-step synthesis, it provides concise and direct routes for your target compounds, streamlining the synthesis process.
Accurate Predictions: Utilizing the extensive PISTACHIO, BKMS_METABOLIC, PISTACHIO_RINGBREAKER, REAXYS, REAXYS_BIOCATALYSIS database, our tool offers high-accuracy predictions, reflecting the latest in chemical research and data.
Strategy Settings
Precursor scoring | Relevance Heuristic |
---|---|
Min. plausibility | 0.01 |
Model | Template_relevance |
Template Set | Pistachio/Bkms_metabolic/Pistachio_ringbreaker/Reaxys/Reaxys_biocatalysis |
Top-N result to add to graph | 6 |
Feasible Synthetic Routes
Disclaimer and Information on In-Vitro Research Products
Please be aware that all articles and product information presented on BenchChem are intended solely for informational purposes. The products available for purchase on BenchChem are specifically designed for in-vitro studies, which are conducted outside of living organisms. In-vitro studies, derived from the Latin term "in glass," involve experiments performed in controlled laboratory settings using cells or tissues. It is important to note that these products are not categorized as medicines or drugs, and they have not received approval from the FDA for the prevention, treatment, or cure of any medical condition, ailment, or disease. We must emphasize that any form of bodily introduction of these products into humans or animals is strictly prohibited by law. It is essential to adhere to these guidelines to ensure compliance with legal and ethical standards in research and experimentation.