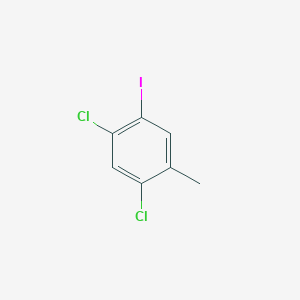
1,5-Dichloro-2-iodo-4-methylbenzene
Overview
Description
1,5-Dichloro-2-iodo-4-methylbenzene is an organic compound with the molecular formula C7H5Cl2I It is a derivative of toluene, where two hydrogen atoms on the benzene ring are replaced by chlorine atoms and one by an iodine atom
Mechanism of Action
Target of Action
The primary target of 2,4-Dichloro-5-iodotoluene A related compound, dcoit, has been shown to have a high affinity for g protein alpha subunits (g α), suggesting the targeted effects on signaling transduction from g protein-coupled receptors (gpcrs) in teleost brains .
Mode of Action
The exact mode of action of 2,4-Dichloro-5-iodotoluene is not well-documented. Given the structural similarity to other halogenated hydrocarbons, it may interact with its targets in a similar manner. For instance, DCOIT’s interaction with GPCRs could potentially alter signal transduction pathways .
Biochemical Pathways
The specific biochemical pathways affected by 2,4-Dichloro-5-iodotoluene Related compounds such as dichlorophenols have been shown to undergo dehalogenation through a distal meta-fission pathway, resulting in the formation of 2-hydroxy-3,5-dichloro-6-oxo-hexa-2,4-dienoic acid .
Pharmacokinetics
The ADME (Absorption, Distribution, Metabolism, and Excretion) properties of 2,4-Dichloro-5-iodotoluene The compound’s molecular weight (28692 g/mol) and its halogenated nature suggest that it may have significant lipophilicity, potentially influencing its absorption and distribution .
Result of Action
The molecular and cellular effects of 2,4-Dichloro-5-iodotoluene The dehalogenation of related compounds can result in the formation of metabolites that may have further biological effects .
Biochemical Analysis
Biochemical Properties
2,4-Dichloro-5-iodotoluene plays a significant role in biochemical reactions, particularly in the context of its interactions with enzymes and proteins. It is known to interact with cytochrome P450 enzymes, which are involved in the metabolism of various xenobiotics. The interaction between 2,4-Dichloro-5-iodotoluene and cytochrome P450 enzymes can lead to the formation of reactive intermediates that may further react with cellular biomolecules, potentially causing oxidative stress and cellular damage . Additionally, 2,4-Dichloro-5-iodotoluene can bind to certain proteins, altering their structure and function, which can have downstream effects on cellular processes.
Cellular Effects
The effects of 2,4-Dichloro-5-iodotoluene on cells are multifaceted. It has been observed to influence cell signaling pathways, gene expression, and cellular metabolism. For instance, exposure to 2,4-Dichloro-5-iodotoluene can activate stress response pathways, such as the mitogen-activated protein kinase (MAPK) pathway, leading to changes in gene expression that help the cell cope with the induced stress . Moreover, 2,4-Dichloro-5-iodotoluene can disrupt cellular metabolism by inhibiting key metabolic enzymes, resulting in altered energy production and metabolic flux.
Molecular Mechanism
At the molecular level, 2,4-Dichloro-5-iodotoluene exerts its effects through several mechanisms. One primary mechanism is the binding to and inhibition of specific enzymes, such as cytochrome P450 enzymes, which are crucial for the detoxification of xenobiotics . This inhibition can lead to the accumulation of toxic intermediates, causing cellular damage. Additionally, 2,4-Dichloro-5-iodotoluene can interact with DNA, leading to changes in gene expression and potential mutagenic effects. The compound’s ability to generate reactive oxygen species (ROS) further contributes to its molecular mechanism of action, causing oxidative damage to cellular components.
Temporal Effects in Laboratory Settings
In laboratory settings, the effects of 2,4-Dichloro-5-iodotoluene have been studied over various time periods. The compound is relatively stable under standard laboratory conditions, but it can degrade over time when exposed to light and heat . Long-term exposure to 2,4-Dichloro-5-iodotoluene in cell cultures has shown that it can cause sustained oxidative stress, leading to chronic cellular damage and apoptosis. In in vivo studies, prolonged exposure has been associated with tissue-specific toxicity, particularly in the liver and kidneys.
Dosage Effects in Animal Models
The effects of 2,4-Dichloro-5-iodotoluene vary with different dosages in animal models. At low doses, the compound may cause mild oxidative stress and transient changes in gene expression. At higher doses, it can lead to significant toxicity, including liver and kidney damage, due to the accumulation of toxic intermediates and ROS. Threshold effects have been observed, where a certain dosage level results in a marked increase in adverse effects, highlighting the importance of dosage regulation in experimental settings.
Metabolic Pathways
2,4-Dichloro-5-iodotoluene is metabolized primarily through the action of cytochrome P450 enzymes . The metabolic pathways involve the formation of hydroxylated metabolites, which are further conjugated with glucuronic acid or sulfate for excretion. These metabolic transformations can affect the compound’s bioavailability and toxicity. The presence of chlorine and iodine atoms in the molecule can influence the metabolic rate and the nature of the metabolites formed.
Transport and Distribution
Within cells and tissues, 2,4-Dichloro-5-iodotoluene is transported and distributed through passive diffusion and active transport mechanisms . The compound can interact with specific transporters and binding proteins, which facilitate its movement across cellular membranes. Its lipophilic nature allows it to accumulate in lipid-rich tissues, such as the liver and adipose tissue, where it can exert its toxic effects.
Subcellular Localization
The subcellular localization of 2,4-Dichloro-5-iodotoluene is influenced by its chemical properties and interactions with cellular components . It is primarily localized in the endoplasmic reticulum, where cytochrome P450 enzymes are abundant. This localization is critical for its metabolic activation and subsequent effects on cellular function. Additionally, 2,4-Dichloro-5-iodotoluene can be found in the mitochondria, where it can induce oxidative stress and disrupt mitochondrial function.
Preparation Methods
Synthetic Routes and Reaction Conditions
The synthesis of 1,5-Dichloro-2-iodo-4-methylbenzene typically involves halogenation reactions. One common method is the iodination of 2,4-dichlorotoluene. This can be achieved by reacting 2,4-dichlorotoluene with iodine and a suitable oxidizing agent, such as nitric acid, under controlled conditions . The reaction is usually carried out in an organic solvent like dichloromethane at a temperature range of 0-25°C.
Industrial Production Methods
Industrial production of this compound may involve similar halogenation processes but on a larger scale. The use of continuous flow reactors and optimized reaction conditions can enhance yield and purity. The choice of solvent, temperature control, and reaction time are critical factors in industrial synthesis .
Chemical Reactions Analysis
Types of Reactions
1,5-Dichloro-2-iodo-4-methylbenzene undergoes various chemical reactions, including:
Substitution Reactions: The iodine atom can be replaced by other nucleophiles, such as amines or thiols, under suitable conditions.
Oxidation Reactions: The methyl group can be oxidized to form corresponding carboxylic acids or aldehydes.
Reduction Reactions: The compound can be reduced to remove halogen atoms, leading to the formation of less substituted toluenes.
Common Reagents and Conditions
Substitution: Reagents like sodium amide or thiourea in polar solvents at elevated temperatures.
Oxidation: Strong oxidizing agents like potassium permanganate or chromium trioxide in acidic or basic media.
Reduction: Reducing agents such as lithium aluminum hydride or hydrogen gas in the presence of a catalyst.
Major Products
Substitution: Formation of 2,4-dichloro-5-aminotoluene or 2,4-dichloro-5-thiolotoluene.
Oxidation: Formation of 2,4-dichloro-5-iodobenzoic acid or 2,4-dichloro-5-iodobenzaldehyde.
Reduction: Formation of 2,4-dichlorotoluene or 2-chlorotoluene.
Scientific Research Applications
1,5-Dichloro-2-iodo-4-methylbenzene has several applications in scientific research:
Biology: Employed in the study of halogenated aromatic compounds’ effects on biological systems.
Medicine: Potential use in the development of new drugs due to its unique halogenation pattern.
Industry: Utilized in the production of specialty chemicals and materials with specific properties.
Comparison with Similar Compounds
Similar Compounds
2,4-Dichlorotoluene: Lacks the iodine atom, making it less reactive in certain substitution reactions.
2,4-Dichloro-5-bromotoluene: Similar structure but with a bromine atom instead of iodine, leading to different reactivity and applications.
2,4-Dichloro-5-fluorotoluene: Contains a fluorine atom, which significantly alters its chemical properties and biological activity.
Uniqueness
1,5-Dichloro-2-iodo-4-methylbenzene is unique due to the presence of both chlorine and iodine atoms on the benzene ring. This combination of halogens provides distinct reactivity patterns and potential for diverse applications in synthesis and research.
Properties
IUPAC Name |
1,5-dichloro-2-iodo-4-methylbenzene | |
---|---|---|
Source | PubChem | |
URL | https://pubchem.ncbi.nlm.nih.gov | |
Description | Data deposited in or computed by PubChem | |
InChI |
InChI=1S/C7H5Cl2I/c1-4-2-7(10)6(9)3-5(4)8/h2-3H,1H3 | |
Source | PubChem | |
URL | https://pubchem.ncbi.nlm.nih.gov | |
Description | Data deposited in or computed by PubChem | |
InChI Key |
RJVCIWMIAJMKGK-UHFFFAOYSA-N | |
Source | PubChem | |
URL | https://pubchem.ncbi.nlm.nih.gov | |
Description | Data deposited in or computed by PubChem | |
Canonical SMILES |
CC1=CC(=C(C=C1Cl)Cl)I | |
Source | PubChem | |
URL | https://pubchem.ncbi.nlm.nih.gov | |
Description | Data deposited in or computed by PubChem | |
Molecular Formula |
C7H5Cl2I | |
Source | PubChem | |
URL | https://pubchem.ncbi.nlm.nih.gov | |
Description | Data deposited in or computed by PubChem | |
Molecular Weight |
286.92 g/mol | |
Source | PubChem | |
URL | https://pubchem.ncbi.nlm.nih.gov | |
Description | Data deposited in or computed by PubChem | |
Retrosynthesis Analysis
AI-Powered Synthesis Planning: Our tool employs the Template_relevance Pistachio, Template_relevance Bkms_metabolic, Template_relevance Pistachio_ringbreaker, Template_relevance Reaxys, Template_relevance Reaxys_biocatalysis model, leveraging a vast database of chemical reactions to predict feasible synthetic routes.
One-Step Synthesis Focus: Specifically designed for one-step synthesis, it provides concise and direct routes for your target compounds, streamlining the synthesis process.
Accurate Predictions: Utilizing the extensive PISTACHIO, BKMS_METABOLIC, PISTACHIO_RINGBREAKER, REAXYS, REAXYS_BIOCATALYSIS database, our tool offers high-accuracy predictions, reflecting the latest in chemical research and data.
Strategy Settings
Precursor scoring | Relevance Heuristic |
---|---|
Min. plausibility | 0.01 |
Model | Template_relevance |
Template Set | Pistachio/Bkms_metabolic/Pistachio_ringbreaker/Reaxys/Reaxys_biocatalysis |
Top-N result to add to graph | 6 |
Feasible Synthetic Routes
Disclaimer and Information on In-Vitro Research Products
Please be aware that all articles and product information presented on BenchChem are intended solely for informational purposes. The products available for purchase on BenchChem are specifically designed for in-vitro studies, which are conducted outside of living organisms. In-vitro studies, derived from the Latin term "in glass," involve experiments performed in controlled laboratory settings using cells or tissues. It is important to note that these products are not categorized as medicines or drugs, and they have not received approval from the FDA for the prevention, treatment, or cure of any medical condition, ailment, or disease. We must emphasize that any form of bodily introduction of these products into humans or animals is strictly prohibited by law. It is essential to adhere to these guidelines to ensure compliance with legal and ethical standards in research and experimentation.